2:I[5784,["173","static/chunks/173-6969cb89628ef0d4.js","297","static/chunks/app/newsroom/page-c45028d2bdade808.js"],"default"]
1b:I[9275,[],""]
1c:I[1343,[],""]
1d:I[5790,["173","static/chunks/173-6969cb89628ef0d4.js","231","static/chunks/231-ab71cbdaacaa8248.js","185","static/chunks/app/layout-94ecf19e137be07b.js"],"default"]
1e:I[8173,["173","static/chunks/173-6969cb89628ef0d4.js","231","static/chunks/231-ab71cbdaacaa8248.js","738","static/chunks/738-e7a03dea596939c7.js","931","static/chunks/app/page-d7219a6fcf502fca.js"],"Image"]
1f:I[231,["173","static/chunks/173-6969cb89628ef0d4.js","231","static/chunks/231-ab71cbdaacaa8248.js","738","static/chunks/738-e7a03dea596939c7.js","931","static/chunks/app/page-d7219a6fcf502fca.js"],""]
3:Ta10,Robert Langer, Sc.D., an Institute Professor at the Massachusetts Institute of Technology (MIT), has been awarded the 2023 Dr. Paul Janssen Award for Biomedical Research. The annual honor goes to a scientist who’s made a transformational contribution toward improving human health.
And “transformational” describes Langer’s work to a T.
Widely recognized as “the father of controlled drug delivery and tissue engineering,” Langer is being honored for his groundbreaking work in designing novel drug delivery systems that can deliver medications continuously, precisely and at controlled rates over extended periods. His pioneering research into biomedical compounds for drug delivery and tissue engineering has impacted a wide range of medical technologies, including anticancer therapy, vaccine development and gene therapy, among others.
“Early in my career at MIT, a number of professors said the whole idea of doing drug delivery didn’t make sense, that it was wrong,” recalls Langer, whose research lab at MIT is considered the largest biomedical engineering lab in the world. “I believed in what I was doing so I just kept trying.” Pushing the boundaries of science to change the trajectory of health
Pushing the boundaries of science to change the trajectory of health
Established in 2004 by what is now called Johnson & Johnson Innovative Medicine (formerly known as the Janssen Pharmaceutical Companies of Johnson & Johnson), the prestigious award celebrates dedicated researchers and champions of science whose basic or clinical discoveries have made, or have the potential to make, a measurable impact on human health. The award honors the legacy of “Dr. Paul,” who helped save millions of lives through his contribution to the discovery and development of more than 80 medicines, four of which remain on the World Health Organization’s list of essential medicines today.
Since its inception, the award has recognized 23 scientists, eight of whom have gone on to win the Nobel Prize for the same work. Winners are chosen by an independent selection committee of the world’s most renowned scientists. The award includes a $200,000 cash prize.
“Any award that celebrates science is a good thing—especially if it can inspire young people and people everywhere to see that science can do good things,” says Langer. “Science can make the world a better place.”
To hear from Langer directly and learn more about his work, watch the video below.4:T2211,“Science is a hard, grueling process, and much of the time quite monotonous. That’s why it’s called ‘re-search’; we do it over and over and over again.” The remark, made during a lively discussion about the arduous work of science and the personal determination that drives it, received a few chuckles from the audience. But it was also meant as a serious take on an underappreciated process. That it was delivered by Nobel Laureate and Janssen Award winner Craig Mello only underscored its import.
On that balmy, late summer evening in 2014, in a stately, yet intimate ballroom at the New York Public Library, Mello was speaking on a panel at the annual Dr. Paul Janssen Award (DPJA) for Biomedical Research ceremony. Named to honor a legendary figure in the field of drug discovery—known by his peers as “Dr. Paul”—the award celebrates the passion and creativity of biomedical innovators with an attendant ceremony that convenes some of the world’s brightest scientists, healthcare industry leaders and journalists. I have been lucky enough to attend many ceremonies over the years and to witness up close the indefatigable spirit that drives these luminaries.
The awardees being honored on that particular night six years ago were Drs. Jennifer Doudna and Emmanuelle Charpentier, for their ground-breaking work on CRISPR Cas9. The gene-editing technology allows for scalable, efficient and precise DNA manipulation, and it has since touched off a revolution in genomics research and applications. It was thus no surprise to anyone when just this past October, the Nobel Committee bestowed the 2020 Prize in Chemistry to Doudna and Charpentier for their efforts.
CRISPR Cas9 has virtually unlimited applications, from eliminating pathogens and supercharging disease research to creating heartier, more nutritious crops. According to Andy Marshall, Chief Editor ofNature Biotechnology, “Transformative is a term rarely heard in biology, but Doudna and Charpentier’s genius was to distill natural CRISPR systems into a simple RNA guide and endonuclease enzyme kit that made gene editing accessible to any research lab across the world. It truly transformed biology and medicine, and continues to do so.”
In other years, Nobel-level recognition of DPJA winners has come even sooner: Craig Mello was named the first winner in 2006, the same year he received the Nobel Prize in Physiology or Medicine, along with Andrew Z. Fire, for his pioneering work in RNA interference (RNAi). Thisancient and long-overlooked biological process helps control gene expression, and it enables organisms to protect themselves from viruses, to adapt to changing environments, and to maintain stable, well-differentiated tissues. While RNAi is a natural cellular process, it is also a potent tool for the exploration and manipulation of gene expression. Mello, insatiably curious about the world since he was a little boy peering through the woods and looking under rocks of his native Virginia, served on the DPJA Selection Committee for several years. His work in RNAi continues to be an important part of biotechnology. “Almost every aspect of our health ultimately depends on how well our body’s cells regulate themselves — or fail to,” notes John Rennie, former editor-in-chief ofScientific American. “Scientists keep finding examples of RNAi fine-tuning the expression of our genes and making sure that our cells stay in good running order. It’s no surprise that it’s become the focus of so much interest as a source of new therapeutics.”
In 2016, Yoshinori Ohsumi, then at the Tokyo Institute of Technology, was honored first with a DPJA in the late spring, and then, only months later, with the Nobel Prize in Physiology or Medicine for his work uncovering the mechanism of autophagy. From the Greek words “auto” meaning “self” and “phagein” meaning “to eat”, this catabolic process eliminates unnecessary or damaged cellular components, making it vital part of cell maintenance. While the process has been known since the early 1960s, the molecular mechanisms of autophagy were deduced by Ohsumi and his team by studying yeast cells and identifying the genes that drive it. He further elucidated the sophisticated machinery that regulates autophagy in human cells, opening a whole new avenue for therapeutic investigation. Mutations in autophagy genes can propel disease, and the autophagic process is involved in several conditions including cancer and neurological afflictions. Notes Rennie on the topic, “While the role of autophagy in cancer is complicated, it’s not hard to believe that someday we’re going to be able to use it to strip malignant cells out of the body more effectively.”
On the subject of cancer, it was the 2018 DPJA winner, Jim Allison, who also took the Nobel Prize in Physiology or Medicine that same year for his discovery that the immune system could be coaxed into fighting tumors, fundamentally altering our oncological arsenal. Cancer finds sophisticated ways to hide from immune attacks, which normally seek out and destroy mutated cells. The fighting foot soldiers of our immune system, T cells, contain a protein, CTLA-4, that acts like a brake to inhibit the immune response.
While other researchers were exploiting the mechanism as a target in the treatment of autoimmune disease, Allison had the opposite idea. He wanted to release the brakes and let the immune response intensify. After developing an antibody that could bind to CTLA-4 and block its function, he proved how this could disengage the T-cell brake and unleash the immune system to attack cancer cells. The first drugs exploiting this principle hit the market in 2011, and their number has grown since.
“Jim Allison’s genius was his continual building of a critical body of evidence to support his thesis that immune checkpoints could be harnessed against cancer, despite strong headwinds from the scientific mainstream,” Marshall says. “But intellectual giants never quite follow the mainstream. They go after big ideas.”
This past fall another very big idea was on display but at a very different kind of DPJA ceremony. With little in-person mingling and a more “digitized” version of scientific camaraderie, the virtual DPJA ceremony still captured the essence of celebration, made all the more poignant in a year in which the world needs biomedical innovation more than ever.
I logged on just in time to watch physician-scientist Susan Desmond-Hellmann, former CEO of the Bill & Melinda Gates Foundation, and Paul Stoffels, Chief Scientific Officer of Johnson & Johnson, pay a quiet, eloquent tribute to this year’s winner, biochemist and cancer researcher Lewis Cantley.
More than 30 years ago, Cantley and his team discovered an enzyme, phosphoinositide 3-kinase (PI3K), and they have been tirelessly pursuing its relation to insulin regulation, cancer and other diseases ever since. Cantley embodies the DPJA spirit. As a young boy growing up in West Virginia, his first foray into science was making homemade fireworks, at the suggestion of his father. “I knew I wanted to be a scientist from very early on,” Cantley remarked in a recent interview, “I was largely influenced by my father who, whenever I asked a question about how things work, would always come up with a logical explanation rather than resort to saying ‘because God made it that way.’”
Cantley’s work implicated PI3K across multiple diseases, and more generally in human cellular metabolism. His insights, as Hellmann noted in her comments at the virtual ceremony, “have made a huge contribution to precision medicine for cancer.” Therapies targeting the PI3K pathway have proliferated over the last 8 years, and have accelerated the march against malignancy.
Summing up the inspiration of this year’s event, Stoffels, beaming on screen as he normally would in person, underscored the reason we celebrate science and scientists like Cantley, particularly in an era where they can help solve one of the biggest health challenges the planet has ever seen: “What inspires me most is the extreme collaboration we are seeing across all groups, scientists, regulators, industry, policy: this collaboration and the passion people are bringing to the current world problems is unprecedented.”
If ever there were a reason and a time for science and scientists to “win”, this is it, in the here and now.
This article has been reposted with permission from Scientific American. Click here to view the original article.5:T9f9,Good evening everyone. As outgoing Chair of the Paul Janssen Award Selection Committee, I am pleased to have a chance to congratulate this year’s awardees.
Let me begin by saying a few words about what the selection committee looks for when we choose laureates. First, and foremost, we look for those who have opened or transformed a field though a bold and seminal discovery. Second we ask if that advance or discovery is likely to have a broad impact on our understanding of biology, physiology and/or medicine. And third we ask if the individual or individuals has been an intellectual leader who has brought positive energy to their field to inspire growth and development. Indeed, these criteria in many ways reflect the legacy of Dr. Paul Janssen.
This year’s laureates fulfill these criteria in spades and their discoveries detailing the machinery and mechanisms of protein folding is remarkable on a number of fronts. First, their work overturned long-standing dogma about how proteins fold in cells, challenging the idea that the linear sequence of a polypeptide is, in and of itself, sufficient to dictate proper protein folding in the cell. Instead, they found that protein machines known as a chaperonins perform this vital task. Second their work has broad implications for all of biology because chaperonins are found throughout living organisms ranging from microbes to man, and in multiple cellular compartments including the cytoplasm and mitochondria. Third, problems in protein folding are associated with a variety of diseases - most notably neurodegenerative disorders such as Alzheimer’s and ALS - and thus the discoveries of our laureates have tremendous potential to yield transformative insights into mechanisms underlying debilitating human disease.
From a basic science perspective, and especially for those of us who are excited by biochemistry and biophysics, chaperonins are just beautiful things to behold. Often described as a capsule or can with a lid that sequesters unfolded proteins until they attain their proper shape, chaperonins are perhaps the loveliest example of how evolution has solved a fundamental problem in a way that is so elegantly revealed by the structure and lifecycle of this magnificent biological machine.
On behalf of the selection committee, I am pleased to congratulate Drs. Hartl and Horwich for their profound and beautiful scientific discoveries and achievements, which are recognized by this year’s Dr. Paul Janssen Award in Biomedical Research.6:T67e,Johnson & Johnson has named Franz-Ulrich Hartl, M.D., Director of the Max Planck Institute of Biochemistry and Arthur Horwich, M.D., Sterling Professor, Yale School of Medicine and Howard Hughes Medical Institute as winners of the 2019 Dr. Paul Janssen Award for Biomedical Research. Dr. Hartl, a biochemist and Dr. Horwich, a geneticist, won for their revolutionary insights into the fundamental molecular process of protein folding.
Proteins are the molecules in cells that drive almost every biological process. Through their collaboration, Hartl and Horwich discovered that proteins are folded into their proper shape with the help of other specialized proteins called molecular chaperones. This process allows the proteins to reach their functional shape, which enables them to perform their biological function. These insights have broad implications throughout biology and medicine, and hold promise for the development of new therapeutic strategies for treating diseases like Parkinson’s, Alzheimer’s and cancer, in which defects in protein folding are involved.
“Drs. Hartl and Horwich combined their brilliant insights and elegant approaches to overturn the dogma of their day about the process of protein folding,” said David Julius, Ph.D., Professor and Chair of the Department of Physiology, University of California, San Francisco, and Chair of the 2019 Dr. Paul Janssen Award selection committee. “Their studies revolutionized our understanding of how proteins achieve their shape and revealed how defects in this process may contribute to a variety of disorders ranging from metabolic to neurodegenerative diseases.”7:T67e,Johnson & Johnson has named Franz-Ulrich Hartl, M.D., Director of the Max Planck Institute of Biochemistry and Arthur Horwich, M.D., Sterling Professor, Yale School of Medicine and Howard Hughes Medical Institute as winners of the 2019 Dr. Paul Janssen Award for Biomedical Research. Dr. Hartl, a biochemist and Dr. Horwich, a geneticist, won for their revolutionary insights into the fundamental molecular process of protein folding.
Proteins are the molecules in cells that drive almost every biological process. Through their collaboration, Hartl and Horwich discovered that proteins are folded into their proper shape with the help of other specialized proteins called molecular chaperones. This process allows the proteins to reach their functional shape, which enables them to perform their biological function. These insights have broad implications throughout biology and medicine, and hold promise for the development of new therapeutic strategies for treating diseases like Parkinson’s, Alzheimer’s and cancer, in which defects in protein folding are involved.
“Drs. Hartl and Horwich combined their brilliant insights and elegant approaches to overturn the dogma of their day about the process of protein folding,” said David Julius, Ph.D., Professor and Chair of the Department of Physiology, University of California, San Francisco, and Chair of the 2019 Dr. Paul Janssen Award selection committee. “Their studies revolutionized our understanding of how proteins achieve their shape and revealed how defects in this process may contribute to a variety of disorders ranging from metabolic to neurodegenerative diseases.”8:T43b,Immunologist James Allison has spent over thirty years studying T cells and developing strategies for cancer immunotherapy. Now, he’s looking at new ways to unleash the immune system to eradicate cancer.
This video was produced for Johnson & Johnson Innovation by Scientific American Custom Media, a division separate from the magazine's board of editors.
When James Allison started researching T cells in the 1980s, he didn’t intend to cure cancer. He was just interested in the immune system. More than thirty years later, his work forms the basis for many of the most promising cancer therapeutics available today. Allison is now the chief immunologist at the University of Texas MD Anderson Cancer Center and the winner of the 2018 Dr. Paul Janssen Award but he still remembers his roots in research. And he’s reaching back to them to drive his immunology revolution forward once again.9:T1ef4,This story originally appeared on jnj.com
We sat down with the winner of the 2018 Dr. Paul Janssen Award for Biomedical Research to learn how his tireless cancer research efforts have helped revolutionize the way we treat the disease and save lives.
Cancer. It's a diagnosis that no one ever wants to hear. But thanks to innovations in treatment, there is more hope than ever before. And one of the most significant breakthroughs in cancer treatment has been immunotherapy, which harnesses a patient's own immune system to fight the disease.
After decades of dedicated oncology and immunotherapy research, James Allison, Ph.D., director of the Parker Institute for Cancer Immunotherapy in San Francisco and the executive director of the Immunotherapy Platform at MD Anderson Cancer Center in Houston, discovered a new way to recruit the immune system to attack solid tumor cancers.
His discoveries around T cell responses garnered him the 2018 Dr. Paul Janssen Award for Biomedical Research, placing him among just 15 other scientists who have won the award since 2006. The award was established to honor the work of Paul Janssen, founder of Janssen Pharmaceutica, by recognizing scientists whose work has helped improve public health.
As the New York City awards ceremony kicks off on September 12, we sat down with Dr. Allison to learn more about his groundbreaking work.
Q: What inspired your career in cancer research?
A:When I was a kid, my father wanted me to become a doctor like him. But when I had the chance to do a summer tutorial and laboratory work as a junior in high school, I got the research bug—and then my interest was further piqued by a course on immunology.
In the class I took, we learned about T cells—a type of white blood cell that protects against infection. Not much was known about T cells at the time, and I was curious to find out more. I figured if we could understand how T cells work, we could discover how to kill cancer cells without the destructive side effects of radiation and chemotherapy.
Plus, cancer was always in the back of my mind for personal reasons. My mother died of lymphoma when I was 10 years old, and two uncles also passed away from cancer when I was young.
I also have a history with the disease: I’ve been diagnosed with prostate cancer and melanoma, which were both caught early. And I'm currently undergoing immunotherapy for early stage bladder cancer.
Q: What makes your research so revolutionary?
A: When I was a kid, my father wanted me to become a doctor like him. But when I had the chance to do a summer tutorial and laboratory work as a junior in high school, I got the research bug—and then my interest was further piqued by a course on immunology.
In the class I took, we learned about T cells—a type of white blood cell that protects against infection. Not much was known about T cells at the time, and I was curious to find out more. I figured if we could understand how T cells work, we could discover how to kill cancer cells without the destructive side effects of radiation and chemotherapy.
Plus, cancer was always in the back of my mind for personal reasons. My mother died of lymphoma when I was 10 years old, and two uncles also passed away from cancer when I was young.
I also have a history with the disease: I’ve been diagnosed with prostate cancer and melanoma, which were both caught early. And I'm currently undergoing immunotherapy for early stage bladder cancer.
Q: What makes your research so revolutionary?
A: In the past, researchers thought the job of T cells was to kill cancer, similar to how chemotherapy works. Early attempts to harness the immune system involved creating therapeutic vaccines derived from cancer cell components to try to get T cells to attack the tumor.
My approach was very different in that it basically ignored the tumor and instead focused on figuring out how to block signals that inhibited the powers of T cells.
Through my research, I discovered a protein on the T cell, called CD28, which acts as its gas pedal, as well as a receptor on the T cell, called CTLA-4, which acts as its brakes. Basically, CD28 and CTLA-4 turn the T cells on and off like a car.
No one realized this braking mechanism needed to be turned off in order for the immune system to unleash a powerful attack on cancer. With this discovery, we were able to figure out how to utilize this switchlike ability to temporarily disable the brakes and create treatments that effectively target cancer.
Q: What has been the most rewarding part of your research?
A: It has been amazing to see how my research has affected the lives of patients.
One stands out in particular: Sharon Belvin was in her early 20s and had metastatic melanoma. Every available treatment to her had failed, so she got involved in early clinical trials of immunotherapy treatments.
It was the last chance she had to survive—it was either that or go into hospice care.
A few months after starting the immunotherapy treatment, she bounced back. One year later, she was declared tumor-free. At the time, I was working at Memorial Sloan Kettering Cancer Center, and saw her after she met with her doctors there. She hugged me and said she had been convinced that her doctors were going to tell her she had relapsed—but the exact opposite had happened.
It's hard to describe the impact this made—she was the first patient I'd met who had been affected by my work.
Q: What does winning this award mean to you?
A: I'm so humbled to get a prize in Paul Janssen's honor. Like me, Paul Janssen was a man of the basic sciences, who studied physics, chemistry and biology before medicine.
I really want people to know the importance of basic sciences and support them, too. Sometimes they get neglected by funding, but new drugs and breakthroughs do come from studying chemistry, biology and physics.
For example, if I hadn't been so focused on the very basic components of the T cell, I never would have made my discoveries that helped revolutionize immunotherapy.
I think that immunotherapy is going to become part of the standard of care for different types of cancer. The next step in my research is finding out why certain patients don’t respond to this treatment. Once we can figure that out, we can greatly improve the quality of this treatment and see even more patients survive.
Meet Sharon Belvin—the metastatic melanoma survivor Dr. Allison mentions—who shares her personal cancer journey, and the amazing impact he had on her life, in this video.a:T52d,Johnson & Johnson has named James Allison, Ph.D., of University of Texas MD Anderson Cancer Center, winner of the 2018 Dr. Paul Janssen Award for Biomedical Research. Dr. Allison won for pioneering a novel and effective strategy to harness the immune system for treating solid tumor cancers. His research over the past four decades has led to breakthrough therapies that are providing hope for patients around the world, and represents a shining example of how fundamental studies of biological processes open the way to the development of transformative therapeutics.
Immunotherapy is treatment that uses a person’s immune system to fight diseases. In the last several decades, it has become an important tool in treating some types of cancer. Dr. Allison is the Chair of the Department of Immunology, the Vivian L. Smith Distinguished Chair in Immunology, Director of the Parker Institute for Cancer Research, and the Executive Director of the Immunotherapy Platform at MD Anderson Cancer Center. He has spent a distinguished career studying the regulation of T cell responses and developing strategies for cancer immunotherapy. His present work seeks to improve immune checkpoint blockade therapies currently used by clinicians and identify new targets to unleash the immune system in order to eradicate cancer.b:T3847,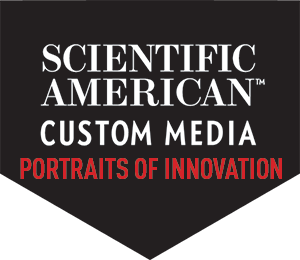
By John Rennie, Science Editor & Journalist
In fiction, scientific breakthroughs are always dramatic. When researchers make a paradigm-shattering discovery, the world instantly hails it as revolutionary. Real life can be slower to hand out rewards. After molecular biologists Victor Ambros and Gary Ruvkun reported on a peculiar exception to the normal rules of gene regulation, it took seven years for the significance of that finding to emerge.
Today, the anomalies discovered by Ambros and Ruvkun and called microRNAs are recognized as the first members of an unexpected world of tiny regulatory molecules that now number in the thousands. These short, single-stranded RNA molecules are about ten times smaller than anyone had thought to look for before Ambros and Ruvkun. They help control a wide variety of processes, both normal and pathological, including embryonic development, differentiation of blood cells, muscle function, congenital heart disease, viral infections, and cancers such as lymphoma and leukemia. Within just a few years, microRNAs have gone from a newly revealed feature of basic biology to a foundation for emerging medical practice.
In recognition of the conceptual and clinical importance of microRNAs, Ambros and Ruvkun have been showered with honors, including Albert Lasker Award for Basic Medical Research, the Gairdner International Award, the Breakthrough Prize in Life Sciences, the Gruber Genetics Prize and, in 2012, the Dr. Paul Janssen Award for Biomedical Research. These acknowledgements are well-deserved and recognize a long-term partnership between the scientists, which started in a laboratory at the Massachusetts Institute of Technology nearly four decades ago.
WHEN VICTOR AMBROS APPLIED TO M.I.T. FOR UNDERGRADUATE STUDY IN 1971, HIS ADMISSION ESSAY WAS JUST SIX WORDS: “I WANT TO BE A SCIENTIST.”
As a boy growing up in Vermont, he had read the biographies of famous researchers avidly, and they spurred in him a desire to become part of that historical tapestry of discovery and invention. Originally, he leaned toward a career in astronomy, in interest his parents had helped along with a kit for building a telescope.But it was biology that Ambros ultimately studied at M.I.T., both during his undergraduate years and then as a graduate student.
Under the Nobel laureate David Baltimore, Ambros worked on the structure of the polio genome. Then, in 1979 he joined the M.I.T. laboratory of Nobel laureate Robert Horvitz as a post-doctoral fellow, where he investigated the genetic regulation of development in the reproductive system of the roundworm Caenorhabditis elegans. (C. elegans has been studied in minute detail for decades, and is still a commonly used animal model for developmental biology and neurobiology research.)
During his work on C. elegans, Ambros uncovered an intriguing relationship between two of its genes, lin-4 and lin-14. Their effects were complementary: mutant worms in which the lin-4 gene was broken or underactive showed the same developmental defects as mutant worms in which the lin-14 gene was overactive. The logical conclusion to draw was that lin-4 normally suppressed the activity of lin-14.
Yet, it was not obvious how to isolate the lin-4 or the lin-14 genes to decode how they worked. To solve the problem, Ambros began to brainstorm with another newcomer to the lab, molecular biologist Gary Ruvkun.
Ruvkun’s journey to that laboratory was more circuitous. Born in 1952 in Oakland, Calif., he became electrified by astronomy, the first NASA spaceflights, and science in general. Like Ambros, he read voraciously and had parents who nourished his passions, with gifts of a telescope and microscope. At the University of California at Berkeley, Ruvkun became fascinated with physics, learning of revolutions on atomic and galactic scales. He also began to learn molecular biology, taking inspiration from the discovery of the double helix and genetic code. He graduated with a bachelor degree in biophysics in 1973.
After a couple of years of working in reforestation in Oregon and traveling throughout Latin America, Ruvkun returned to academia at Harvard in 1976. He took up the study of recombinant DNA technology in the laboratory of Frederick Ausubel, who was interested in engineering crop plants with the nitrogen-fixing ability found in some bacteria. In his PhD research, Ruvkun identified the genes that fix nitrogen in these bacteria and discovered that they are conserved over vast evolutionary distances. His focus on evolutionary conservation is a recurrent theme in his microRNA research as well. After earning his doctorate, Ruvkun also did research in Nobel laureate Walter Gilbert’s lab at Harvard, and brought his molecular genetics expertise to the Horvitz lab at M.I.T. In the Horvitz lab, Ruvkun and Ambros developed an approach to allow the molecular characterization of C. elegans genes, including lin-14 and lin-4.
Ruvkun and Ambros teamed up to investigate the interaction of lin-4 and lin-14, but by 1984, when they were each leaving to start their own labs—Ambros at Harvard and Ruvkun at Massachusetts General Hospital and Harvard Medical School—they had not yet found the answer. They agreed to keep working on the problem, with Ambros focusing on lin-4 and Ruvkun on lin-14. Cloning and characterizing the lin-4 gene was a painstaking process.
Ambros credits the arrival of Rosalind Lee in his lab as a technician in 1987 as a turning point. Lee (who had also worked in the Baltimore lab and had married Ambros in 1976) could devote the time and effort to the tedious job of cloning lin-4. She and post-doctoral fellow Rhonda Feinbaum worked for the next four years on the project, until they had finally identified lin-4’s sequence. Contrary to expectations that lin-4 might encode a small regulatory protein, they found instead that lin-4 makes a noncoding string of RNA only 22 nucleotides long, which in its precursor form folds back on itself like a hairpin.
During this same period, Ruvkun determined that the part of the lin-14 gene that is negatively regulated by lin-4 mapped to a region of the messenger RNA that is not translated into protein. And he discovered that this region was highly conserved in evolution.
As the exact RNA sequences of lin-4 and lin-14 emerged in the Ambros and Ruvkun labs, Ambros and Ruvkun decided to compare the sequences of their two genes to see if the model that these RNAs base paired with each other would be supported.
ONE EVENING, THEY READ OFF THE SEQUENCES OF NUCLEOTIDES TO EACH OTHER ON THE TELEPHONE AND WERE ASTONISHED TO SEE THAT PORTIONS OF LIN-4 AND LIN-14 WERE COMPLEMENTARY, BUT NOT PRECISELY COMPLEMENTARY—THERE WERE SURPRISING LOOPS AND BULGES IN THE RNA DUPLEXES, BUT DUPLEXES NEVERTHELESS—A FACT THAT SUGGESTED AN AMAZING, UNORTHODOX POSSIBILITY.
Years earlier, molecular biologists had observed that some plants and bacteria fight off viral infections by deploying single-strand RNA molecules against them. These RNAs are described as antisense because they are complementary to sense sequences in the viral genes. By binding to viral gene transcripts, these RNAs somehow blocked the production of viral proteins.
Ambros and Ruvkun realized that something similar, but distinct with its bulges and loops and incredibly tiny length, could be going on between lin-4 and lin-14: very small RNA transcripts of lin-4 could be suppressing the expression of the lin-14 gene. The evolutionary conservation of both the lin-4 and the lin-14 RNA segments pointed to this base pairing, as well as the RNA bulges and loops, as being under evolutionary selection. Biotechnologists were already experimenting with using antisense RNA to shut down genes artificially. Perhaps nature had evolved some similar mechanism for silencing genes in a complex cell, too. And perhaps the design of perfect RNA duplexes was too simplistic and too long; nature had simply evolved more intricate and interesting forms of regulatory RNAs that Ambros and Ruvkun were the first to see.
After confirming their suspicion, Ambros and Ruvkun each wrote a paper about their discovery, and those papers were published back-to-back in the journal Cell in 1993.
Remarkable as this news was and despite the prominence of its publication, other scientists’ response to it was muted. Even Ambros and Ruvkun were unsure whether this bizarre regulatory mechanism was anything more than an oddity of the worm. As Ambros reflected in his acceptance speech for the Lasker Award (subsequently published as an essay for Nature Medicine in 2008), “Why was I so pessimistic about the prospect of lin-4 being the harbinger of a diverse class of regulatory molecules of broad importance? … There was no theoretical need to explain existing phenomena in terms of new mechanisms or new classes of molecules.” The traditional model of gene regulation involving protein transcription factors generally still worked fine.
Worse, Ambros and Ruvkun could not find equivalents to the lin-4/lin-14 microRNA system in other species distant from roundworms, so the mechanism might be irrelevant to other organisms. “Despite decades of model system genetics and gene cloning, no other example of a small RNA gene product like lin-4 had been identified. So, maybe lin-4 really was a peculiarity of Caenorhabditis developmental timing mechanisms,” Ambros wrote. However, Ruvkun observed in his acceptance speech for the Lasker Award (also published in Nature Medicine 2008), that there were precedents from RNA biology, for example, the ribosomal RNA, that are universal to all life, so that the lin-4/lin-14 paradigm could be general.
In 2000, miRNAs emerged from their corner of biology. Ruvkun discovered a second microRNA in C. elegans, let-7.
HE ALSO SHOWED THAT LET-7 IS CONSERVED THROUGHOUT THE ANIMAL KINGDOM, FROM HUMANS TO INSECTS, WHICH PROVED THAT MICRORNAS WERE A WIDELY USED REGULATORY MECHANISM, NOT JUST A FLUKE.
In his Lasker Award speech, Ambros, who had not known about Ruvkun’s let-7 in-all-animals project, recalled being stunned by its significance: “After reading their paper in the autumn of 2000, I had to set aside 10 minutes to stare out the window and reorganize my view of the universe.” At about the same time, another RNA-based mechanism of gene regulation, RNA interference, was discovered and shown to be mediated by RNAs of the same diminutive size as the miRNAs of Ambros and Ruvkun. The biologists Andrew Fire and Craig Mello first showed that C. elegans can use double stranded RNA to silence other genes, and called the process RNA interference. In 2006 that work brought Fire and Mello a Nobel prize and brought Mello a Janssen Award. Then David Baulcombe, a plant geneticist at Sainsbury Laboratory in U.K., showed that plants generate tiny antisense-acting RNAs during RNA interference that were roughly the same size as lin-4 and let-7.
Interest in microRNAs took off. Today, a search for journal articles about microRNA turns up more than 59,000 citations—more than 52,000 of them since 2010. Cancer research is only one area in which microRNAs have risen to prominence. MicroRNAs generally seem to be less abundant in tumor cells, which suggests they may normally help to rein in the development of malignancy. The distinctive microRNA signature of individual tumor cells might therefore be useful in flagging the origins of a patient’s cancer and in identifying which courses of treatment might be most effective. MicroRNAs can circulate in the blood and other body fluids, which makes them easy targets for medical diagnostic products. The company Mirna Therapeutics is developing a cancer treatment, currently in clinical trials, based on replacing the microRNAs deficient in abnormal cells. Outside oncology, microRNAs could also one day find a wealth of clinical applications in the treatment of neurodegenerative illnesses and other conditions because they seem to influence the developmental plasticity of stem cells.
Ambros continues to be a leader in the study of microRNAs. In his laboratory at University of Massachusetts Medical School, which he started in 2008, he explores the complexities of gene regulatory networks and the role of microRNAs with them. One focus of those studies is how microRNAs help to buffer organisms against physiological and environmental stresses to improve organisms’ survival.
Ruvkun remains very active in microRNA and siRNA research. His laboratory has studied how microRNA and siRNA genes regulate their targets and has developed genetic tools to increase the effectiveness of these regulatory RNAs. Ruvkun explores other aspects of biology as well. For example, he discovered that C. elegans controls its metabolism and lifespan through a signaling pathway that depends on an insulin-like receptor molecule, just as mammals do. Beyond its practical value for suggesting new targets for future diabetes drugs, this discovery implies that the molecular pathways governing metabolism and longevity have been strongly conserved through hundreds of millions of years of evolution, a recurrent theme in his research.
Ruvkun and his colleagues are also developing techniques that could detect so-called extremophile microorganisms that might play an overlooked role in some human disease. A spinoff of that project is literally out-of-this-world: for over 20 years, Ruvkun has worked on analytical systems that future robot landers on Mars could use to search for evidence of life that shares ancestry with life on Earth. The effort is called the Search for Extraterrestrial Genomes Project (SETG). Again, Ruvkun is searching for deep conservation of biology, in this case between planets.
READINGS Gitschier J. In the Tradition of Science: An Interview with Victor Ambros. PLoS Genet. 2010; 6(3): e1000853. doi:10.1371/journal.pgen.1000853 Nair P. Profile of Gary Ruvkun. PNAS. 2011; 108 (37) 15043-15045. doi:10.1073/pnas.1111960108 © Scientific American 2017c:Tc46,Dame Carol Robinson, DBE, FRS, FMedSci Doctor Lee’s Professor of Chemistry, University of Oxford 2017 Dr. Paul Janssen Award Selection Committee Member 23 June 2017
The mitochondrion is often described as the powerhouse of the cell, controlling cellular respiration and producing ATP. While the origins of this vital machinery are not fully understood, we now know one thing for sure - that our mitochondrial DNA comes from our mother. Douglas Wallace first demonstrated this inheritance by experiments on human mitochondrial DNA, extracted from blood platelets, donated by independent families. Using restriction enzymes, he showed that in each family the progeny had the maternal cleavage pattern. He concluded: ‘Only a maternal mode of inheritance is in complete agreement with the data’.1
Long before I knew who was responsible for this discovery, I was struck by its significance. Primarily, as it is counter-intuitive – all DNA had previously been considered to come from both parents. It must have taken great courage to propose.
How does this arise and why is this inheritance line so special? Unlike all other genetic material that is passed along with chromosomes in our cells, mitochondrial DNA comes from the mother’s egg. Roughly 200,000 mitochondria are present in the egg while sperm have far fewer – those that enter the egg are destroyed rapidly. As a consequence, our mitochondria, and their DNA (mtDNA), are inherited exclusively from our mothers
Wallace did not simply sit back and contemplate his discovery. Instead, he realised its implication for the construction of phylogenetic trees. His reasoning was straightforward: chance mutations accumulate in a piece of DNA at a roughly constant rate and within each generation a new mutation will occur. The DNA of two distinct lines will, therefore, show a number of changes proportional to the time that they shared a common ancestor. By sequencing mtDNA of populations around the world, it is possible to determine the relative amount of time that it has been since each of them shared a common mother. Reconstructing the maternal ancestry revealed that humans arose in Africa approximately 200,000 years ago.
The significance of his discovery goes beyond informing our ancestral heritage. Understanding mitochondrial disease with its frequently observed traits such as muscle weakness, diabetes, hearing loss and impaired vision, has profound implications for the many sufferers. While mitochondrial transfer treatments are still in their infancy, developments in gene editing and epigenetics increase the chances of finding alternative cures.
The winner of this year’s Dr. Paul Janssen Award lives up to the high standards of innovation and ingenuity embodied by all previous recipients. Moreover, Douglas Wallace will inspire the next generation of scientists to embrace the unexpected. When results are surprising, or appear at first to be wrong, dramatic new insight can be gained, as ably demonstrated by this year’s awardee.
Reference 1. Proc. Natl. Acad. Sci. USA 77, 6715-6719, 1980.d:Ta4d,By Seema Kumar
06/2017
Science impacts our daily lives in every imaginable way. From antibiotics and vaccines, to personalized medicine, wearable devices and 3D printing, science has improved the human condition, leading to longer, healthier, happier lives for people all over the world. But science can also seem complex and shrouded in mystery. It can be hard to make the connection between research in a lab and the impact it has on our everyday lives.
That’s why science needs champions.
Science needs champions to help us understand how research and innovation can create a better future for all of us. To celebrate the heroes of science whose insatiable curiosity and tenacity results in positive impact for society. To make science simple, human.
And the time to champion science is now.
To fuel progress and deliver the next generation of treatments and cures, to help achieve a world without disease, to help all of us live longer and healthier lives, each of us can be a champion of science.
At Johnson & Johnson, we are proud to celebrate and fuel scientific innovation through the work that we do every day, and through supporting programs like The Dr. Paul Janssen Award for Biomedical Research and Biotechnology Institute’s BioGENEius Challenge, to celebrate today’s pioneers and inspire tomorrow’s leaders.
Join us in creating more champions of science!
Use and follow #ChampionsofScience to show your support. For every use of #ChampionsofScience, we will donate $5, up to $50,000, to the Biotechnology Institute through September 30 to engage and empower the next generation of scientific innovators .
For more information about the #ChampionsofScience movement and our commitment to fueling scientific and technological breakthroughs for today and the future, visit PaulJanssenAward.com.
e:T4102,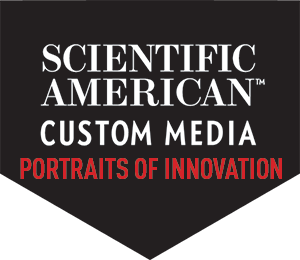
By John Rennie, Science Editor & Journalist
Modern biotechnology dawned in the early 1970s when researchers first began to cut and splice the DNA of living things to endow them with desirable new genetic traits. Since then, biotechnologists have pushed ahead to many victories, but that success did not come easily. Compared to the ambitions that scientists and entrepreneurs have had for it, genetic engineering has often been costly and slow to execute, uncertain in its results, and impractical for use on some organisms.
Meanwhile, nature’s simplest organisms quietly guarded a superior way to edit genomes, which they first evolved as a defense hundreds of millions of years ago. The recent discovery of that secret, and its development into a dazzlingly superior method of genome engineering generally known as CRISPR, has electrified the life sciences in less than a decade—and challenged the scientific community to use it wisely. And although CRISPR is not without controversies, none can dispute the singular importance of biologists Emmanuelle Charpentier and Jennifer Doudna in bringing it to light. For that exceptional work, they were named as the winners of the Dr. Paul Janssen Award for Biomedical Research in 2014.
Born outside Paris in 1968, Emmanuelle Charpentier was always encouraged in diverse academic and artistic pursuits by her parents. She shared her mother’s interests in psychology, but she also favored philosophy, mathematics, and especially medicine. She vowed early in life to make a contribution to people’s medical well being.
Charpentier pursued undergraduate study of microbiology, genetics, and biochemistry in Paris at University Pierre and Marie Curie (UPMC) between 1986 and 1992. The life of a researcher suited her, she learned: the science nourished her curiosity about nature’s secrets and she enjoyed working as part of a collaborative team. She continued with graduate work at Institut Pasteur (1992-1995) and at UPMC (1993-1995), during which she studied the molecular mechanisms underlying antibiotic resistance. It also led her to the related study of how bacteria interact with their surroundings during the process of infecting hosts.
“Along my career, I have always developed tools to improve the precision of genetics studies, whether in bacteria or mice” Charpentier explains.
Stints as a post-doctoral fellow took her to Institut Pasteur (1995-1996), Rockefeller University (1996-1997), New York University’s Langone Medical Center (1997-1999), St. Jude Children’s Research Hospital (1999) and the Skirball Institute of Biomolecular Medicine (1999-2002). She then returned to Europe as the head of a laboratory at Vienna University, in its Institute of Microbiology and Genetics. Charpentier had been focusing her work on how bacteria sometimes use small RNA molecules to regulate the expression of their genes. In Vienna, that interest led her to think about new discoveries that were just being made about a peculiar feature in the genomes of many bacteria (and other ancient cells named archaea): odd clusters of repeating nucleotide sequences punctuated by short spacers of DNA seemingly stolen from viruses. Bacterial geneticists called them clustered regularly interspaced short palindromic repeats—or more conversationally, CRISPR.
Microbiologists’ hypothesis in the early 2000s was that CRISPR was a piece of something like an immune system in bacteria that defended them against viruses to which they (or their ancestors) had been exposed. For the bacteria, CRISPR was like a wall of genetic wanted posters for known viral threats. But details of how CRISPR enables this defense were a mystery when Charpentier first began to think about it. With her colleagues, Charpentier quietly began to dig into the problem. They identified specific small RNA molecules associated with CRISPR that interacted with a DNA-slicing enzyme called Cas9. Charpentier suspected that these RNAs, which included bits of the viral gene sequences, guided Cas9 to targets in viral DNA and allowed it to destroy viruses infecting the cell. The concept was unorthodox because, hitherto, only complexes of proteins guided by RNA were known to perform that kind of DNA-targeting function in cells.
Further experiments, completed after Charpentier moved to Umeå University in 2009, established important proof for her bold idea. She showed that when CRISPR RNA containing viral sequences is transcribed, it matures by forming a duplex with another bit of RNA (called tracrRNA) and then binding to Cas9. The resulting complex seemed to have all the pieces needed to thwart a viral attack. Charpentier first presented her discovery at a conference in 2010, and then more formally in a paper in Nature in 2011.
Already it was clear to her that if the CRISPR-Cas9 system could locate DNA targets in cells so quickly and efficiently, it held immense potential as a tool for genetic engineering. Yet to realize that possibility, she needed more detailed, structural understanding of how the system worked.
The needed expertise presented itself a few months later, when Charpentier attended a conference in Puerto Rico and met Jennifer Doudna, an eminent structural biologist and Howard Hughes Medical Institute investigator at the University of California in Berkeley. Doudna, too, had been studying RNAs involved in the CRISPR system for several years. Unlike Charpentier, Doudna had not started off in life imagining a scientific career for herself. Yet, growing up on the Big Island of Hawaii, she had been fascinated by the extraordinary variety and adaptations in the natural world around her. At Pomona College, she studied biochemistry. In 1985, she moved on to graduate work at Harvard University with Jack Szostak, who had pioneered the study of how early RNA molecules with the right chemical properties might have kicked off life’s evolution. In his biochemistry laboratory, Doudna could concentrate on developing novel ribozymes—RNA molecules that have catalytic chemical properties like enzyme proteins.
In 1991 she headed to the University of Colorado at Boulder and the laboratory of Thomas Cech, who had recently shared a Nobel prize for the discovery of ribozymes. There, she began working on crystallizing and learning the three-dimensional structure of a particular ribozyme (at that time, scientists knew the folded structure of only one other RNA molecule). She continued that project after joining the faculty of Yale University in 1994, and eventually succeeded in 1996. The experience only cemented Doudna’s conviction that knowledge of RNA structures was essential to understanding their function. When the opportunity to have her own laboratory at the University of California, Berkeley, arose in 2000, Doudna took it in part because she would have handier access to the synchrotron x-ray source at Lawrence Berkeley National Laboratory, which would help with the crystallization studies.
At Berkeley, Doudna had turned her attention to CRISPR-Cas systems, intrigued by many of the same questions that had drawn in Charpentier. When she met Charpentier at the conference in Puerto Rico in 2011, they hit it off personally and also recognized that they brought complementary strengths to the study of CRISPR-Cas. Notwithstanding the huge gulf between their laboratories—Doudna’s on the West Coast, Charpentier’s in Vienna—they vowed to collaborate.
The fruit of their joint effort was their epic paper in Science in August 2012, which showed that the stored viral sequence in a mature CRISPR RNA duplex does indeed direct its associated Cas9 enzyme to slice up the corresponding viral DNA whenever and wherever it manifests in a cell.
Yet the paper did more than explain how bacteria use CRISPR to defend themselves against viruses. It also showed that researchers could use tailored RNA to program CRISPR-Cas9 complexes to slice a DNA double helix wherever they wanted. In conjunction with a cell’s DNA-repair mechanisms, they could delete a gene or insert a new one. Moreover, CRISPR’s usefulness for genome regulation may be at least as important as for genome editing. With CRISPR, scientists can introduce programmable transcription factors to cells’ DNA. With those, they can reversibly silence or deactivate specific genes without altering them. As Doudna noted during a TED talk in 2015, “We realized that we could harness its function as a genetic engineering technology—a way for scientists to delete or insert specific bits of DNA into cells with incredible precision—that would offer opportunities to do things that really haven't been possible in the past.”
Genome researchers and biotechnologists have rushed to use the new CRISPR technique. With every passing year, the number of published papers citing the Charpentier-Doudna paper has increased exponentially.
Charpentier and Doudna have continued to improve on the technique, as have other researchers including Feng Zhang of the Broad Institute at the Massachusetts Institute of Technology and George M. Church of Harvard (who were each independently working on developing CRISPR for genome modification at the same time as Doudna and Charpentier).
Several biotech start-ups have jumped in to commercialize applications of CRISPR technology, including CRISPR Therapeutics (co-founded by Charpentier), Caribou Biosciences and Intellia Therapeutics (both co-founded by Doudna), and Editas Medicine (co-founded by Doudna, Zhang and others. The intellectual property rights surrounding CRISPR are currently in dispute: a U.S. patent on the technique was initially granted to Feng Zhang and the Broad Institute, but Doudna and Charpentier objected that they had filed for a patent first; an investigation into who should hold the rights is now pending with the U.S. Patent and Trademark Office. Nevertheless, the technique remains freely available for use by academic researchers around the world, and they are making the most of it.
Already, agricultural researchers are applying CRISPR to engineer pest- and disease-resistant wheat, rice, oranges, soybeans and other crops. Animal researchers have been making disease-resistant pigs, and ones with “humanized” organs that might be safe donors for transplants to human beings. Biomedical investigators used it to treat mice with a condition resembling Duchenne muscular dystrophy. Entomologists are exploring the possibility of altering the genetics of wild mosquito populations to reduce their ability to spread malaria and Zika virus.
CRISPR is also of intense interest to medical researchers as a means of treating heritable illnesses such as cystic fibrosis and sickle cell disease, as well as DNA-infiltrating ones like HIV. It is widely believed that within a decade a CRISPR-based therapy may be tested against a human illness—possibly a blood disease in adults, since blood cell lines are easier to manipulate than most tissues are, and non-heritable genetic alterations to adults are less ethically fraught.
Acclaim and prestigious honors for Charpentier and Doudna have been abundant because of CRISPR. In addition to the Janssen Award, they shared the 2015 Breakthrough Prize in Life Sciences, the 2015 Gruber Prize in Genetics, and the 2016 Canada Gairdner International Award (along with Feng Zhang), among many others. Charpentier received the Leibniz Prize of the German Research Foundation in 2016 and was elected as a member of the Royal Swedish Academy of Sciences. (Doudna is also a member of the National Academy of Sciences, but she was elected to that body in 2002 on the strength of her ribozyme structural work.) Charpentier and Doudna are widely regarded as likely recipients of a Nobel Prize in the near future.
Today, in her Berlin laboratory as a director at the Max Planck Institute for Infection Biology, Charpentier continues to study bacterial CRISPR systems and to work on further refinements of the gene editing technology that sprang from it. But she has also returned to work on some of the other broad mysteries in biology that have always interested her, such as the interactions between bacteria and their hosts and the molecules that govern that relationship at the genetic and biochemical level.
Doudna, meanwhile, has become extremely involved in the ongoing international discussion about how to apply CRISPR wisely. As she and other scientists have warned, enthusiasm for the technique should not blind anyone to the risks of unintended consequences. Treating genetic conditions in ailing body tissues like muscle sounds sensible; the almost irresistible temptation to alter human embryos or germline tissues for disease prevention in future generations should not be indulged lightly. Ethicists worry about CRISPR being used too casually to “improve” humans by eliminating conditions that are not strictly pathological, and that altered DNA passing on to future generations—a situation that could be a boon or a eugenic nightmare. Ethicists worry about the eugenic nightmares that could come from “improvements” made to the DNA of future generations. Eugenicists worry that benign efforts to eliminate genetic illnesses from the human population permanently could too easily slide into the eugenic nightmare of “improving” humans by deleting traits that are not pathological, merely unpopular.
For that reason, Doudna has helped to spearhead a movement among scientists to discuss and govern CRISPR technology. In November 2015, an international assembly of them met and over three days worked out a set of accords that declared a temporary, voluntary moratorium on most potential CRISPR experiments on humans. Under the terms of that agreement, experiments on human ova would proceed only if the potential benefits of an experiment greatly exceeded the potential harm, and only on human embryos that had no possibility of being brought to term.
“I am excited about the potential for genome engineering to have a positive impact on human life, and on our basic understanding of biological systems,” Doudna wrote in a commentary for Nature in December 2015, adding, “But I also think that today's scientists could be better prepared to think about and shape the societal, ethical and ecological consequences of their work.”
READINGS
Abbott A. The quiet revolutionary: How the co-discovery of CRISPR explosively changed Emmanuelle Charpentier’s life. Nature. 2016; 532:432–434. doi:10.1038/532432a
Achenbach J. Pondering ‘what it means to be human’ on the frontier of gene editing. The Washington Post. 2016 May 03.
Deltcheva E, Chylinski K, Sharma CM, Gonzales K, Chao Y, Pirzada ZA, Eckert MA, Vogel J and Charpentier E. CRISPR RNA maturation by trans-encoded small RNA and host factor RNase III. Nature. 2011; 471:602-607. doi:10.1038/nature09886
Doudna J. How CRISPR lets us edit our DNA. TED Talk transcript. 2015.
https://www.ted.com/talks/jennifer_doudna_we_can_now_edit_our_dna_but_le...
Jinek M, Chylinski K, Fonfara I, Hauer M, Doudna JA and Charpentier E. A programmable dual-RNA-guided DNA endonuclease in adaptive bacterial immunity. Science. 2012; 337:816-21. doi:10.1126/science.1225829
Ledford H. Alternative CRISPR system could improve genome editing. Nature. 2015; 526:17. doi:10.1038/nature.2015.18432
Lewis R. A Conversation with CRISPR-Cas9 Inventors Charpentier and Doudna. DNA Science Blog. PLOS Blogs. 2015 Dec 03. http://blogs.plos.org/dnascience/2015/12/03/a-conversation-with-crispr-c...
Marino M. Biography of Jennifer A. Doudna. PNAS. 2004; 101(49):16987-16989. doi:10.1073/pnas.0408147101
Pollock A. The Gene Editor. The New York Times (NY edition). 2015 May 11. D1.
Reardon S. Welcome to the CRISPR zoo. Nature. 2016; 531:160–163. doi:10.1038/531160a
Johnson CY. Control of CRISPR, biotech’s most promising breakthrough, is in dispute. The Washington Post. 2016 Jan 13.
© Scientific American 2016 f:Tb06,David Julius, Ph.D., Professor and Chair of the Department of Physiology at the University of California, San Francisco, and Chair of the 2017 Dr. Paul Janssen Award selection committee
As the call for nominations for the 2017 Dr. Paul Janssen Award gets underway, I am honored to be part of the independent selection committee who has the privilege and responsibility to select the next esteemed Award winner. The Award has an impressive record, having recognized 14 outstanding researchers since 2004, two of whom went on to win the Nobel Prize. Who will be next?
For me, being part of the selection committee is exciting because it gives me an opportunity to delve into ground-breaking science that is taking place all over the world, and learn about the scientists who are leading inspirational and breakthrough discoveries. Whether in academic, government or company labs, researchers all over the globe are passionately pursuing answers to new challenges, pushing boundaries of our understanding, and uncovering mechanisms that may one day change the way we manage the most pressing health issues facing us today.
While most of us do not pursue a career in research for the accolades, I believe that it is important to celebrate the significant role that scientific discovery plays in advancing human health. Scientific awards like the Dr. Paul Janssen Award remind us that basic science and advances in clinical medicine are inseparably intertwined, and innovative scientific research at every level is crucial to furthering our understanding of the mechanisms underlying human health and disease.
Prizes also help increase awareness outside of the lab of the value of scientific innovation to society. They serve as inspiration for the future of scientific research and discovery, and encourage the next generation of innovators to be bold and passionate in their work. And they remind us that scientific curiosity and achievement is key to our cultural and intellectual growth, no less so than art, music, and literature.
The Dr. Paul Janssen Award was established by Johnson & Johnson to honor the legacy of Dr. Paul Janssen, one of the most impactful pharmaceutical scientists of the 20th century, and to celebrate today’s outstanding scientists. In the spirit of Dr. Janssen’s reputation for curiosity, innovation, integrity, passion, and leadership, the independent selection committee, which I am honored to chair this year, looks for awardees whose contributions embody these important attributes.
If you know a scientist whose discoveries have paved new scientific or therapeutic ground, have inspired your own research, or have positively impacted the lives of others, please take a few moments to submit a nomination! They may be our next winner!10:Te4e,By Paul Stoffels, M.D., Chief Scientific Officer, Johnson & Johnson
09/2016
The long road to scientific discovery starts with a question. For Dr. Paul Janssen, that question was “what’s new?” This was the basic inquiry - and profound challenge - that he posed to the scientists who worked in his lab, a reminder to always continue innovating medicines to meet the needs of patients who are waiting eagerly for new solutions.
It was a simple question that led to the discovery and development of an astounding 80 new medicines and made a difference for millions of patients.
Dr. Paul was the founder of Janssen Pharmaceutica, which became part of the Johnson & Johnson Family of Companies in 1961. He was also one of the most innovative pharmaceutical researchers of our time and a true inspiration. I had the incredible fortune to learn from and work alongside Dr. Paul. I was always struck by his compassion for human suffering, which fueled his lifelong commitment to finding new and better medicines and treatments for patients.
Dr. Paul’s vibrant legacy continues to inspire us every day at Johnson & Johnson to find innovative medicines and products that make a difference for patients. To keep this spirit alive, we celebrate the outstanding achievements of today’s scientists with the prestigious Dr. Paul Janssen Award for Biomedical Research. Over the past decade we’ve recognized a remarkable roster of scientists. While their discoveries are vastly different, these scientists have one thing in common: they each embody the leadership and passion of Dr. Paul.
In a magnificent library overlooking Central Park, we recognized the 2016 Dr. Paul Janssen Award winner, Dr. Yoshinori Ohsumi, during an awards ceremony at the New York Academy of Medicine in New York City. It was the perfect setting to celebrate Dr. Paul’s profound impact on healthcare and honor Dr. Ohsumi’s contributions toward advancing human health.
Dr. Ohsumi is a cell biologist at the Tokyo Institute of Technology who made pioneering discoveries in autophagy – a basic function of all living cells. When Dr. Ohsumi began his research more than 25 years ago, little was known about the significance of autophagy. His curiosity led him to make discoveries which have opened up a rich and exciting field of research. Dr. Ohsumi’s work is important because it holds promise for helping us better understand, prevent and treat some of the most challenging diseases facing patients today, including cancer and Alzheimer’s. Dr. Ohsumi’s passion for scientific inquiry reminds me of the innovative spirit that Dr. Paul brought to the lab every day.
At Johnson & Johnson, we want to continue to celebrate accomplished scientists like Dr. Ohsumi while also nurturing and encouraging the next generation of scientists. We believe that a great idea can come from anywhere in the world, and with the right support, new ideas can lead to meaningful advances in human health. There are bright innovators inquiring "what's new?" in laboratories around the globe, and we are proud to support, celebrate and collaborate with these great scientists to make a difference in the world.
Dr. Paul Stoffels is Executive Vice President, Chief Scientific Officer Johnson & Johnson. In this role, he works with R&D leaders across Johnson & Johnson to set the enterprise-wide innovation agenda and is a member of the Johnson & Johnson Executive Committee. 11:Tced,The power of basic science
08/2016
Society assigns immense value to the work of professional athletes and entertainers, while scientists’ life-changing discoveries can garner little fanfare. The Dr. Paul Janssen Award for Biomedical Research, established by Johnson & Johnson to honor the legacy of its namesake scientist, recognizes these unsung heroes, who make significant, transformative contributions toward improving public health.
Dr. Ohsumi shares his views on autophagy, the future of the field, and the power of basic science to propel medical innovation.
WHAT MOTIVATED YOU TO BECOME A BIOLOGIST?
When I began my undergraduate studies at the University of Tokyo, I was interested in chemistry. It was the 1960’s and the field of molecular biology was just emerging. It was such an exciting time and I was drawn to explore the molecular machinery that drives basic biological processes.
WHY IS AUTOPHAGY IMPORTANT?
Our bodies make around 400 grams of protein every day, but we only take in from food sources about 70 or 80 grams. The difference comes from pre-existing proteins inside our bodies, which are broken down into their component parts and assembled into new proteins. This recycling process is essential for life, and autophagy is one of the main pathways for degrading proteins.
HOW DID YOU END UP STUDYING AUTOPHAGY?
Not many people were interested in the dynamics of protein turnover in the cell. But our bodies must balance the needs of protein synthesis with the supply derived from protein degradation. I figured this process must be important, so I decided to pursue it.
YOU ONCE SAID YOUR APPROACH TO SCIENTIFIC RESEARCH IS TO LOOK FOR NEW, EVEN UNPOPULAR TOPICS TO STUDY. IF YOU WEREN’T EXPLORING AUTOPHAGY, WHAT WOULD YOU WORK ON?
I am a cell biologist, so many events that unfold within cells interest me. Although I have worked on autophagy for 27 years, I remain fascinated by it — there are so many fundamental questions yet to be answered, even in model organisms like yeast. That information is essential to understand how defects in autophagy can contribute to major human diseases, such as neurodegeneration, cancer, and infection.
HOW DO YOU FEEL ABOUT RECEIVING THE 2016 DR. PAUL JANSSEN AWARD?
I am incredibly honored. When I first began studying autophagy, I never thought it would become such a big and important field. I am very pleased and also thankful for the recognition of the role that basic science plays in driving medical innovation.
WHAT ADVICE WOULD YOU OFFER TO YOUNG SCIENTISTS JUST BEGINNING THEIR CAREERS?
Scientists across the world face enormous pressure and competition for stable funding. Young scientists in particular may choose to work only in popular fields, thinking that path will be less risky and more likely to advance their careers. But to keep the wheels of biomedical progress turning, we must open entirely new fields of research — and attract young scientists to push them forward.12:T1255,By Dr. Hans Clevers, M.D. Ph.D.
06/2016
Sixty years ago, Christian de Duve, an iconic 20th century cell biologist from Belgium, discovered the lysosome. Lysosomes serve as intracellular trash cans, in which cells can dispose of all kinds of biological debris taken up from the outside. Some years later he noted that cells can also discard parts of themselves into these little demolishing machines, presumably to remove worn-out structures and to recycle their building blocks. He coined the term "autophagy" for the process by which cytoplasmic constituents are transported to and degraded in the lysosome. These observations have appeared prominently in the high-school textbooks ever since, but how exactly cells would selectively cannibalize little bits of themselves remained an enigma for several decades. This somewhat esoteric question was taken up 25 years ago by Yoshinori Ohsumi, a scientist at the Tokyo Institute of Technology and now in his seventies.
Ohsumi realized that yeast cells and human cells go about many daily challenges in much the same way, even if the common yeast-human ancestor may have lived more than a billion years ago. Since free-living yeast cells are quite a bit simpler than our own cells, he reasoned that yeast might represent just the right system to address his question. As a first crucial discovery, he noted that yeast cells employ autophagy –they literally start eating themselves- when starved of nitrogen. He then performed a genetic screen to dissect the autophagic process. In other words, he found mutant yeast strains in which the autophagy process was disturbed in one way or another. With the explosion of molecular technology, it was not too difficult to find out which genes were mutant in these strains: He thus rapidly found 15 autophagy-related genes, now called ATG genes. With these genes in hand, he then started working out how they –in concert- allow a yeast cell to mark, transport and digest small intracellular components.
The first striking discovery revealed that a biochemical process that rose to prominence in the 80s, the ubiquitin conjugation system, kick-starts autophagy. Working together with a lipid conjugation system, it builds specialized vesicles (‘autophagosomes’) that have the unique property to engulf intracellular components for delivery to and destruction by de Duve’s lysosome. With these and other discoveries, Ohsumi unveiled almost all components of the autophagy pathway and defined the principles by which these components work together to remove and recycle the right cellular component at the right time. A new field was born. His junior co-workers Noboru Mizushima and Tamotsu Yoshimori as well as others around the globe went on to demonstrate that mammalian cells use the same autophagy genes as yeast – exactly as predicted by Ohsumi.
Now, autophagy is recognized as much more than 'just' a mechanism for turnover of cell parts. It is a central player in some of the most important health threats of current times. Autophagy is a crucial mechanism to survive periods of starvation, from yeast to mammals, while its role in the opposite phenomenon –obesity- is intensively scrutinized. Cells also have learned to utilize the autophagy system to remove unwanted bacterial visitors. The list of these party crashers is impressive and includes tuberculosis, salmonella, Shigella, Coxiella and Listeria, responsible for some of the most frightful infectious diseases on our planet. The role of autophagy in neurological afflictions such as Alzheimer’s and Parkinson is also intensively investigated. Indeed, in one rare hereditary form of Parkinson’s, the gene Parkin is mutated. In healthy cells, Parkin is essential for the autophagy of worn-out mitochondria, the miniature power plants inside cells.
We scientists build on work from others. We are trained to work in established fields. We add details, find new links. We correct mistakes in our own work or in that from others. A rare breed of scientists is different. They are individuals who start new fields, open new horizons. They are the giants on whose shoulders we stand. There are not many. Yoshinori Ohsumi, the winner of this year’s Dr. Paul Janssen Award, is one.
Hans Clevers, M.D. Ph.D., is professor of molecular genetics at the Hubrecht Institute and Research-Director of the Princess Máxima Centre for Paediatric Oncology. Dr. Clevers is also part of the Dr. Paul Janssen Award Selection Committee. 13:T3e5e,
By John Rennie, Science Editor & Journalist
The late Paul Janssen—“Dr. Paul” to much of the world of pharmaceutical research—loved to ask, “What’s new?” For him, it was no casual opening to small talk. The world cried out for medical cures and treatments, and Janssen recognized that only a tenacious pursuit of novel molecules with therapeutic promise would yield them. The chemists in his employ knew it, too: when he regularly posed his favorite question to them, it was both a cheerful query and a firm challenge to keep turning up new observations, new ideas, and new leads.
Thanks to that drive and the heights to which it pushed the company he headed, Janssen is widely considered the most prolific drug inventor of all time. He and the research teams he headed at Janssen Pharmaceutica (and the earlier versions of that company) are credited with discovering more than 80 new active compounds of interest. Some of those medications led to breakthroughs in psychiatric treatment, pain management and anesthesia, the treatment of parasitic and infectious diseases, and a host of other medical specialties. Five of those medications are on the current WHO list of essential medicines. At the time of his death in 2003, he held more than 100 patents and was a listed author on more than 850 scientific publications. He was the recipient of more than 80 medical prizes and held honorary doctorates and memberships in dozens of organizations.
“He respected courage as much as intelligence and experience,” wrote Paul J. Lewi, a longtime colleague, with Adam Smith in a 2007 article about Janssen’s approach to research for R&D Management. That courage Janssen inspired in others, they added, pushed them to “excelling beyond limits that they themselves had thought unattainable.”
When Paul Adriaan Jan Janssen was born in 1926 in Turnhout, Belgium, his father, Constant, was a successful general practitioner who dreamed of being an entrepreneur. Shortly later, Constant struck a deal to import and distribute pharmaceuticals from Gedeon Richter, a Hungarian manufacturer, into Belgium, its colony in Congo, and the Netherlands. By 1934 he was operating the company as N.V. Produkten Richter, and in 1938 he gave up his medical practice to concentrate on the flourishing trade in vitamins, organ extracts, and other traditional medications.HIS FOUR-YEAR-OLD SISTER DIED OF TUBERCULAR MENINGITIS…HER LOSS MADE HIM DECIDE TO DEVOTE HIS LIFE TO MEDICAL RESEARCH”
His father’s example undoubtedly shaped young Paul’s eventual career choice, but a tragedy inspired it more. When Paul was in high school, his four-year-old sister died of tubercular meningitis—a condition that was almost untreatable in that essentially pre-antibiotic time. Her loss made him decide to devote his life to medical research, as he often later explained.
Despite the Nazi occupation of Belgium during World War II, Janssen managed to enroll secretly at the Faculté Universitaire Notre Dame de la Paix in Namur, where he applied himself to learning chemistry, biology, and physics. He continued with medical studies at the Catholic University of Leuven in 1945. For six months in 1948 he traveled in the United States to tour current drug research facilities and to acquaint himself with then state-of-the-art procedures, then returned to Europe and the University of Ghent, from which he graduated with honors as a general medical practitioner in 1951.
Despite the Nazi occupation of Belgium during World War II, Janssen managed to enroll secretly at the Faculté Universitaire Notre Dame de la Paix in Namur, where he applied himself to learning chemistry, biology, and physics. He continued with medical studies at the Catholic University of Leuven in 1945. For six months in 1948 he traveled in the United States to tour current drug research facilities and to acquaint himself with then state-of-the-art procedures, then returned to Europe and the University of Ghent, from which he graduated with honors as a general medical practitioner in 1951.
Two years of obligatory military service then followed, during which Janssen still managed to work as an assistant in illustrious pharmacology laboratories at the University of Cologne and the University of Ghent.
In 1953 Janssen could have joined any number of research laboratories, but he was instead determined to start his own. A powerful idea had taken root in his thinking: that the chemical structures of compounds directly related to their physiological effects, and that by systematically modifying promising molecules one could efficiently find new, desirable synthetic drugs. The legendary Paul Ehrlich, the Nobel laureate inventor of treatments for syphilis and other diseases, had pioneered this medicinal chemistry approach decades previously but Janssen knew its promise had barely been scratched.
Returning to Turnhout, Janssen asked his father for a loan of 50,000 Belgian francs and workspace on the third floor of the family business. He and four assistants then set to work on a highly focused program of developing medically important drug compounds quickly, because Janssen recognized doing so would be a financial necessity if his new venture was to survive and remain independent.
Within a year they had synthesized about 500 compounds; within three years, more than 1,100—an astonishing rate of productivity. The fifth compound they created, ambucetamide, proved to be highly effective as an antispasmodic for treating menstrual pain, and it became the first big winner for Janssen’s young company when it hit the market in 1955. Other important drugs born from those early compounds included isopropamide, a treatment for peptic ulcers and gastrointestinal pain, the anti-diarrheal drug diphenoxylate, and the painkiller dextromoramide, all of which propelled Janssen’s laboratory to fame.
By 1957, the success of Janssen’s company—then called the N.V. Research Laboratorium Dr. C. Janssen—led him to expand and move it to the campus of a former military base in Beerse. There, Janssen realized two of his greatest and longest-lasting pharmaceutical contributions.
The first, created in 1958, was the neuroleptic haloperidol, which helped to revolutionize the treatment of schizophrenia and other psychotic disorders. Through the first half of the 20th century, the prospects for people suffering from schizophrenia had altered little from the era of physical restraint in Victorian madhouses. The introduction of the drug chlorprozamine in the 1950s had helped to change that, but its heavy sedative effects were unsatisfactory for many. Janssen’s haloperidol reduced the symptoms of psychosis without making patients drowsy. It eventually became the most commonly prescribed of the drugs called typical antipsychotics and is still used widely around the globe.
Anesthesia and the treatment of pain were similarly transformed by Janssen’s development of fentanyl in 1960. Fentanyl is a fast-acting, short duration opioid roughly 100 times as potent as morphine but less likely to cause nausea, which makes it almost ideal as a surgical anesthetic. Because fentanyl can also be delivered to the body in a variety of ways, including a slow-release transdermal patch, it has become the most widely prescribed analgesic drug in the world for the treatment of moderate to severe pain, particularly chronic pain from cancer. Janssen oversaw the creation of many other successful analgesics in the fentanyl family, including the anesthetic sufentanil, which is 1,000 times as potent as morphine and particularly well-suited for lengthy surgeries.
Janssen’s meteoric successes did not escape the attention of the wider pharmaceutical industry. A major turning point arrived in 1961, when Janssen agreed to sell his family’s companies to Johnson & Johnson, with the guarantee that his research group would continue to operate independently as Janssen Pharmaceutica. That partnership helped feed the feverish productivity of Janssen’s research enterprise, which continued unabated.
Janssen’s colleagues, friends, and rivals have frequently speculated about the secret to his extraordinary productivity. Part of it, surely, was his devotion to Ehrlich’s medicinal pharmaceutical approach and the systematic strategies he employed to explore the properties of new compounds. Pharmaceutical discovery may always depend on an element of luck, but Janssen understood how to improve the odds in his favor—and the importance of keeping his focus on genuinely therapeutic compounds, not just interesting chemistry. As Sir James Black of King’s College London wrote in a personal appreciation of Janssen in 2005, “As far as I know, Dr. Paul never started a project without a conception in his head, a conception that not only specified a chemical starting place, a ‘lead’ molecule, with appropriate bioassays but also embodied foresight of how his invention would deliver clinical utility.”
When reading, listening, and thinking about chemical concepts, Janssen frequently drew models of molecules by hand on scraps of paper; he believed that doing so helped him build a deeper understanding and intuition about the chemical structures. Sir John Black recalls, “I often watched him at meetings, when bored with the proceedings, finding solace inside his head as he doodled new chemical compounds!”
Still, Janssen was just one man, and the ascendance of Janssen Pharmaceutica came from the efforts of many researchers there. His management style was at least as important a part of his genius as his chemistry instincts were.
By all accounts, Janssen disliked ungainly hierarchies and bureaucratic inefficiency. He therefore favored a much more horizontal organizational chart for his research groups and encouraged the leaders of laboratories to operate independently and creatively. As for his own role, “I am the conductor of an orchestra,” Janssen liked to say.
Lewi and Smith noted that Janssen typically started his day with a briefing from the coordinator of research on the latest data from the labs, after which he toured from lab to lab to hear more about the most interesting results. Near the conclusion of each interview, Lewi and Smith wrote, “it was not unusual for a scientist to take a spoon or something and give a few brief taps on the tubes of the central heating system as a warning sign for those working further down the corridor: take care; be prepared; he’s coming; don’t get taken by surprise and see to it that you have ‘something new’ ready at hand.”
As Theodore Stanley, Talmage D. Egan, and Hugo Van Aken explained in a 2008 article for Anesthesia & Analgesia: “Paul Janssen was unique in building the research of his company around his people, not the other way around. He gave all his coworkers the greatest possible latitude in their area of expertise, while he determined the general direction it would go. He acted firmly but also with great respect for the qualities of his associates. He had the talent of being able to stimulate and encourage them by continually nurturing their hopes.”THINK LIKE A SCIENCE FICTION WRITER AND THEN USE YOUR SCIENTIFIC SKILLS TO REALIZE THAT FUTURE.”
Janssen drove himself to turn up new ideas no less relentlessly than he did his staff. Lewi and Smith recount that Janssen was constantly scanning pharmaceutical and chemistry journals, taking notes on what caught his attention and compiling these thoughts into an in-house newsletter. He also relentlessly followed up on his questions with colleagues around he world. “There was no place for scientists to hide and avoid exposure to the question ‘What’s new?’” Lewi and Smith recalled.
Throughout his lifetime, Janssen oversaw and directed the expansion of Janssen Pharmaceutica’s product line and activities. New initiatives of special relevance to health in developing nations pursued treatments relevant to parasitology, mycology, and neurology. Departments for veterinary medicine and plant protection were added in 1964 and 1972 respectively. These efforts yielded a host of drugs for combating parasitic worms, molds, diarrhea, nausea, and psychoses. A global network of subsidiaries grew up around the world, including a history-making facility in the Shaanxi province of China in 1985, which was the first Western pharmaceutical factory to be established there.
The Shaanxi factory led serendipitously to Janssen making an important contribution to the preservation of Chinese culture. Not far from where the Janssen factory was being built, archaeologists were uncovering the now famous “army” of terracotta soldier statues that had been sculpted to guard the 2,200-year-old mausoleum of the emperor Qin Shi Huang. When Janssen visited the site a decade later, he saw that statues were newly infested with mold that thrived in the heat and humidity. Janssen brought samples of the mold back to his laboratory in Beerse, where his researchers identified the fungi and developed water-based chemical sprays for eliminating it from terracotta. Those treatments helped to save the terracotta army, and went on to help conserve other important historical artifacts throughout China.
In 1995, Janssen and Lewi co-founded the Center for Molecular Design, where they investigated and developed anti-HIV compounds including the diarylpyramidines. One of Janssen’s most cherished hopes was to develop a successful treatment for multi-drug resistant tuberculosis, a highly elusive goal. That work came to fruition in 2012 when the U.S. Food and Drug Administration approved bedaquiline, a compound developed by Janssen Pharmaceutica researchers, as part of a compound therapy for adult TB patients. Approval in the European Union followed two years later.
Paul Janssen did not live to see that success; he died unexpectedly at age 77 on November 11, 2003, during a visit to Rome. He was survived by his wife, Dora, their five children, and 13 grandchildren—but also by an unmatched record of achievement in pharmaceutical science. During his lifetime, Janssen’s accomplishments earned him dozens of scientific and medical prizes and honorary degrees. In 1990 King Baudouin of Belgium elevated him to the peerage by conferring a barony on him.
The long list of drug treatments to his credit and the pharmaceutical research company that bears his name are both monuments to his singular genius. Yet the tribute that might best honor the innovative spirit of the man is the Dr. Paul Janssen Award for Biomedical Research, which Johnson & Johnson founded in his memory in 2005 to “promote, recognize and reward creativity in biomedical research.”
READINGS
Black J. “A Personal Perspective on Dr. Paul Janssen,” J. Med. Chem. 2005, 48:1687-1688. doi: 10.1021/jm040195b
Lewi PJ and Smith A. “Successful Pharmaceutical Discovery: Paul Janssen’s Concept of Drug Research,” R&D Management 2007, 37:355-361. doi: 10.1111/j.1467-9310.2007.00481.x
Stanley TH, Egan TD, and Van Aken H. “A Tribute to Dr. Paul A. J. Janssen: Entrepreneur Extraordinaire, Innovative Scientist, and Significant Contributor to Anesthesiology,” Anesth Analg. 2008 Feb,106(2):451-62. doi: 10.1213/ane.0b013e3181605add.
© Scientific American 201614:Tc9c,By William N. Hait, M.D., Ph.D.
07/2015
In the past two decades, oncology research has transformed our approach to treating patients affected by cancer. Scientists are now able to sequence entire cancer genomes within an individual patient, which has resulted in more precise and effective treatments.
This approach and the advances that ensued are the result of a massive investment in cancer research conducted within universities, research institutes and biotechnology and pharmaceutical companies throughout the world. A notable leader in this effort is Dr. Bert Vogelstein. He and his colleagues formulated the idea that cancer is caused by sequential mutations of specific genes and used human tissues to test this hypothesis. By elucidating the molecular basis for certain forms of cancer, Dr. Vogelstein has advanced our understanding of how cancer develops, and has provided the basis for a great deal of the work that we now refer to as personalized or precision medicine. In fact, when investigators uncover sequential changes in the progression from normal to malignant, they often refer to this as a “Vogelgram”.
Dr. Vogelstein and his team identified the genes responsible for hereditary colorectal cancers, leading to unprecedented insights into the genetic and biochemical events that initiate solid tumors. For these and numerous other achievements, Dr. Vogelstein has received many honors, and most recently has been named the winner of the 2015 Dr. Paul Janssen Award for Biomedical Research.
Dr. Paul was fond of asking his research staff a daily question, “What’s new?” In seeking to challenge the status quo, he motivated those with whom he worked through a steadfast commitment to science and an unmatched intellectual tenacity. Dr. Vogelstein’s research, drive and passion are the embodiment of Dr. Paul’s vision to fearlessly pursue our understanding of disease and to develop novel solutions to improve and extend life for patients.
I have had the privilege of knowing Bert since our days at the University of Pennsylvania, where he not only excelled academically but also as a member of the Penn tennis and squash teams. His work set the standard for cancer research, moving away from the study of immortal cell lines to the deep interrogation of human tissues.
Cancer continues to challenge researchers from academia and industry to pursue new ways of thinking and new avenues of inquiry as we seek to develop solutions for the 14 million new patients affected by cancer diagnosis each year. We at Janssen are committed to building on the work of Dr. Vogelstein, who showed us that the origins of cancer begin many years before the onset of disease and provides us with the tools to intercept cancer before it ever becomes an illness.
Empowered by scientific breakthroughs, spurred on by researchers like Dr. Vogelstein, we enter a new era of hope and promise for cancer patients.
William N. Hait, M.D., Ph.D. is the Global head of Janssen Research and Development, LLC. 15:T1aa5,By Michael S. Brown, M.D.
09/2015
Dr. Brown shared the 1985 Nobel Prize in Physiology or Medicine with his long-term scientific partner Joseph Goldstein. The prize recognized the discoveries that they made with regard to the regulation of cholesterol metabolism
As a senior scientist, I have much experience with prizes. I have served on several prize juries and I have been fortunate to receive a number of prizes together with my scientific partner Joe Goldstein. Sometimes I am asked, “What is the purpose of scientific prizes? Do they benefit science or just the few scientists who receive them?” Here are my views on science prizes.
Let’s begin with two things that science prizes are not. First, they are not goals. They differ from Olympic Medals or Super Bowl Rings. In sports, the goal is to win the prize. If you don’t finish first, you are a loser. In science the goal is to discover truth – prizes are a pleasant after-thought.
Some scientists confuse science with sports. They choose a trendy, competitive scientific field, and they plan their experiments with an eye on the prize. “If I can solve the next problem in this field, I am sure to win a (fill in the blank) prize.” These scientists compete for the notoriety of publishing in “high profile” journals where their work will be widely viewed and may even elicit a laudatory commentary. They flock to repetitive scientific meetings with the same attendees where they expand their contacts and profile. I pity these outwardly driven scientists. Lacking an internal reward system, they depend entirely on the approval of others. Even if they make a fundamental discovery they consider themselves failures if their discovery is not recognized with prizes.
Other scientists are inwardly driven. They are motivated by a passion to answer a question that burns inside of them. Even if no one else considers the question important, the inwardly-driven scientist cannot rest until he or she obtains the answer. The answer invariably leads to more questions, so inwardly-driven scientists pursue the problem into ever deeper layers. Eventually they reach a fundamental level whose ramifications extend much further than the original problem. Recent examples include the scientists who became fascinated with odd repeat sequences in bacterial genomes, later to find that they constituted the bacterial immune system – work that led to the CRISPR-Cas9 technology for gene manipulation in mammals. Other inwardly directed scientists studied the circadian rhythm of pupal hatching in fruit flies, the reproductive practices of hermaphroditic roundworms, or the strange basis of fluorescence in jellyfish – all work that led to profound and general biologic insights.
Scientific prizes are not declarations of genius, despite their public perception. Most prizes recognize the discovery – not the discoverer. The selection committee first decides whether a certain discovery is sufficiently significant to merit a prize. Then they try to identify the scientist(s) who made the discovery. Contrary to popular belief, the jury makes no statement about the intelligence, or even the creativity, of the discoverer. They state simply that he or she has contributed to an important discovery. Admittedly, the average prize-winning scientist is somewhat above average in intelligence when compared with other scientists, but few are near the top. The Nobel Foundation maintains a book of signatures by all previous Nobel laureates. Signing the same book as Albert Einstein does not make one an Einstein.
Complicating the interpretation of their significance, some prizes limit the recipients to three in any single category. The Nobel Foundation initiated this practice many years ago. As scientific fields have expanded, many of the most revolutionary discoveries result from the culmination of efforts by many scientists. In such cases the prize cannot be awarded. Sometimes it is delayed until some of the contributors die, reducing the eligible number to three. For scientific prizes, longevity is a prerequisite.
If competition for prizes creates false motives for scientists and false idols for the public, why do we have them? In my opinion, prizes are beneficial because they show the world that society values scientific discovery. When the President of the United States welcomes American Nobel laureates to the White House, the gesture elevates scientists to nearly the same status as NCAA basketball champions. The resulting publicity may inspire young people to realize that science is a valued endeavor. Of course, we must temper this ambition by explaining that they must first discover something. Prizes are the dessert, not the entrée.
Scientific prizes are beneficial because they immortalize the name of the grantor. Without his prize, Alfred Nobel would barely be remembered as the inventor of dynamite, and Albert Lasker would be the man who coined the phrase “Quaker Oats: Shot from Guns.” The Dr. Paul Janssen Award pays lasting tribute to one of the great pharmaceutical innovators whose discoveries benefitted millions.
When prize juries do their homework carefully they grant public recognition to scientists who discover truth, even the inwardly directed scientists who labored in obscurity. By the time a discovery gains widespread recognition many scientists will have made contributions. The jury must dig through the published literature to identify the few scientists whose initial observations created the field. Frequently the key figure will be an inwardly directed scientist whose work was compelled only by a search for truth. The contributions of this pioneer may have been lost in the swirl of activity that follows a fundamental discovery. Many people will have inserted bricks into the scientific edifice. Prize juries must see past the laborers to identify the architect, and then they must tell the whole story of how the building was conceived and built.
On balance, I believe that prizes are beneficial to science and to the public that relies on science to solve the many problems facing us. The limitations of prizes must be appreciated by the scientists who earn them and the public who applauds them. Let us hope that society continues to reward the pioneers of science.
Michael S. Brown, M.D. is Paul J. Thomas Professor of Molecular Genetics and Director of the Jonsson Center for Molecular Genetics at UT Southwestern. Additionally, he is the chairman-elect of the Dr. Paul Janssen Award for Biomedical Research Selection Committee. 16:Tecd,By Bert Vogelstein, M.D.
09/2015
I am very pleased and honored to be the winner of the 2015 Dr. Paul Janssen Award for Biomedical Research. This award is particularly meaningful to me for a variety of reasons. One of them relates to something my oldest son, Jacob, taught me. Jacob is a biomedical engineer and when I used to show him some of our papers, he would say “Hey, that’s pretty interesting, but you know, just about everything is interesting to someone; there’s a big difference between interesting and useful.”
That perspective, I think, is appropriate for many types of biomedical research, and one of the reasons that this award is unique – because it recognizes the dual importance of what’s useful as well as what's interesting. By interesting, we, as scientists, mean something that has some inner beauty, some aesthetic quality. By useful we mean something that is useful to society, not just to other scientists.
There are very few people who have done both interesting and useful things. One of them is Dr. Paul Janssen. What most impressed me about Dr. Janssen was his long term view. He focused on what might be useful in 15 or 20 years, which is dramatically different from many industry perspectives, which focus on the near term. I believe that’s why Janssen was able to develop so many new drugs.
Two other physician scientists who achieved that duality are Mike Brown and Joe Goldstein. Their work is particularly important to me not only because it epitomizes useful and interesting, but also because they played a major role in charting the course of cardiovascular research over the last decades - and its focus on prevention. Cardiovascular research is centered on primary and secondary prevention, while cancer research is largely devoted on the opposite end of the spectrum - curing advanced disease. The research course that Brown, Goldstein, and others in their field charted has diminished deaths from cardiovascular disease by 75% over the last 60 years. Cancer deaths have decreased hardly at all during the same time period, so there is a lot that we cancer researchers can learn from them.
Another reason that the Janssen Award is meaningful to me is because it’s given by industry. It's often much harder to do something useful, really useful, than to do something that’s just interesting, and what industry scientists do is useful stuff. This award gives me a chance to acknowledge the importance of the work that they do, and to thank them for transforming the interesting stuff that we do in academia into useful products for society.
Finally, based on lessons drawn from industry, the award celebrates collaboration. Industry scientists work in teams. Imitating their approach, I work in a team. My team is co-led by Ken Kinzler, and has included nearly 200 technicians, pre-doctoral and post-doctoral fellows over the last 35 years. These individuals have been responsible not just for doing the vast majority of the experiments in our laboratory but also for devising many of the ideas that led to the experiments as well as the technical advances that made the experiments successful. This award is a tribute to their efforts as much as mine, and makes it all the sweeter.
Bert Vogelstein, MD is the Clayton Professor of Oncology and Pathology at John Hopkins University, the co-director of the Ludwig Center for Cancer Genetics & Therapeutics at the John Hopkins Kimmel Cancer Center, and an investigator at the Howard Hughes Medical Institute. Dr. Vogelstein was the winner of the 2015 Dr. Paul Janssen Award. 17:T120d,By Rebecca Richards-Kortum Ph.D.
01/2016
The Dr. Paul Janssen Award for Biomedical Research honors scientists whose work has led to significant and transformational advances that improve human health. Scientific discoveries made by Janssen Award recipients have led to new and improved treatments for arthritis, cancer, diabetes, and HIV/AIDS. As we celebrate the achievements of the recipients, it is important to ask what the scientific community can do to ensure a robust pipeline of future innovators.
Can Innovation Be Taught? I believe innovation is a skill that can be taught. I’ve seen college freshmen become skilled and confident inventors in less than one semester. Just like science, innovation is a systematic process – it is simply the process of translating scientific discoveries into practical tools designed to address unmet societal needs. And just as we teach students to follow the scientific method, we can teach them to carry out the process of innovation.
Yet, science is often taught as a collection of facts rather than as a process. When students see only the results and not the process that leads to innovation, it is easy for them to believe that innovators are rare individuals born with some special innate ability. As a consequence, many highly capable students who could become successful innovators drop out of science at an early age.
How to Teach Innovation:The best and perhaps only way to teach innovation is to engage students in the process of innovation. The revolution in low-cost and open-source rapid prototyping tools has greatly facilitated efforts to teach innovation (e.g. http://printrbot.com, https://www.arduino.cc , https://www.raspberrypi.org).
For example, students use Rice University’s maker space to develop technologies that improve neonatal survival in sub-Saharan Africa ( http://www.sciencemag.org/content/336/6080/430.summary). Design challenges come from clinicians practicing in low-resource settings, and multi-disciplinary teams of physicians, engineers, and scientists mentor the students as they develop their technologies. Using this collaborative model, Rice students have designed more than 100 novel technologies; several of them have been commercialized and implemented at the country level where they have changed the standard of care, and more are in the pipeline.
When they are given real-world challenges and the tools to brainstorm, prototype, and evaluate solutions, students experience the value of science in action and begin to see themselves as inventors. Emily Johnson, an undergraduate Bioengineering student who participated in a design-based course and implementation internship in Africa, reflected, “When I got to Malawi to demonstrate the pulse oximeter I had worked on all semester at Rice, my perspective changed. Seeing patients in need of a technology gave me motivation to invent a better solution—it wasn’t a proof-of-concept, or just a grade—it was real people needing diagnosis, and I knew I could make an impact.”
Why Teach Innovation? Last June, the White House hosted the nation’s first Maker Fair (https://www.whitehouse.gov/nation-of-makers), calling on educators to dramatically expand the number of students that have the opportunity to become makers. Our data show that students who have opportunities to participate in authentic scientific research and design experiences are much more likely to persist in science - and the effects are greatest for women and under-represented minority students.
Now is the time for scientists and innovators to join with science educators to ensure that we teach science in a way that supports a future generation of Janssen awardees.
Rebecca Richards-Kortum is a Professor at the Malcolm Gillis University’s Department of Bioengineering. She is also the Director for Rice 360° at Rice University’s Institute for Global Health and a member of the Dr. Paul Janssen Award Selection Committee 18:T2784,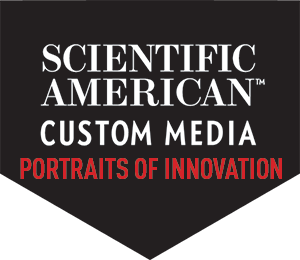
By John Rennie, Science Editor & Journalist
When the molecular biologist Craig C. Mello was a boy in Virginia in the 1960s, he was a disappointing student in the classroom. But outside, left alone to indulge his curiosity, the scientist he would eventually become sprang to life. Whenever possible he spent hours wandering through the woods, peering at leaves and animals, turning over rocks in search of wonders beneath them. His father, a paleontologist who worked for the U.S Geological Service and late the Smithsonian Institution, often brought the family with him on fossil-hunting expeditions to the American southwest, as well as on hikes and camping trips closer to home. Those trips awakened an early love of nature in Mello and a deep appreciation of how prehistoric surprises could survive into the modern world.
How fitting, then, that as a molecular biologist Mello ushered in a revolution in biomedical research with the discovery of an ancient, long-overlooked mechanism that cells use to control gene expression. He and its co-discoverer, Andrew Z. Fire, dubbed it RNA interference, or RNAi, and it appears to be centrally important for enabling organisms to protect themselves from viruses, to adapt flexibly to changing environments, and to maintain stable, well-differentiated tissues. So significant was their finding that in 2006—just eight years after its publication—they were jointly rewarded with the Nobel Prize for Physiology or Medicine, and Mello received the inaugural Dr. Paul Janssen Award for Biomedical Research.
BIOLOGICAL MECHANISMS ARE FAR MORE STABLE THAN THE POSITIONS OF CONTINENTS AND OCEANS ON THE FACE OF THE EARTH, AND COULD EVEN OUTLIVE THE EARTH IF WE FIND A WAY TO COLONIZE SPACE…
As Mello wrote in the autobiography he provided to the Nobel committee, "The biological mechanisms at work inside our cells are truly ancient and remarkably stable, more stable even than the positions of continents and oceans on the face of the Earth."
Mello's boyhood belief that he would become a scientist became irrevocable in the late 1970s when he heard the news that scientists had cloned the human gene for the hormone insulin into bacteria, making it possible to produce limitless supplies of what had been an expensive therapeutic compound. Fascinated with the promise of molecular biology, Mello enrolled at Brown University to study chemistry, which he followed up with further doctoral work at the University of Colorado and Harvard University.
It was during his graduate studies that Mello focused his attention on the nematode Caenorhabdytis elegans. A tiny, transparent soil roundworm with neither a respiratory nor a circulatory system, C. elegans is only a millimeter long and about 1,000 cells in size. Primitive though it might seem, it is exquisitely well-suited to studies of animal development. Investigators have been able to document how every one of those 1,000 cells differentiates and takes up its location in the adult animal. (In 1998 C. elegans also became the first multicellular animal to have its entire genome sequenced.)
During his graduate studies, Mello began to collaborate with Andrew Fire at the Carnegie Institute for Science in Baltimore on a way to insert genetic information—a technique called DNA transformation—into C. elegans. They eventually succeeded and that discovery became the basis for Mello's doctoral thesis in 1990. Mello then moved to the Fred Hutchinson Cancer Research Center in Seattle to continue his studies of the genetic regulation of nematode development. In 1994 he moved to the University of Massachusetts Medical School.
Mello, Fire, and their colleagues experimented with controlling genetic expression in C. elegans by injecting the animals with single strands of so-called antisense RNA—sequences of ribonucleic acids that were complementary to the messenger RNA (mRNA) molecules transcribed from active genes. In effect, antisense RNA turned off genes by binding to their mRNA and prevented it from being translated into proteins.
But Mello, Fire, and other researchers working with C. elegans also noticed that injecting single strands of sense RNA—ones with sequences identical rather than complementary to mRNA molecules—could also selectively shut off genes, which was highly unexpected. And a further, bigger surprise awaited Mello and Fire when they injected paired strands of RNA into the roundworms: the double-stranded RNA was vastly more potent at censoring a matching gene than the single strands were. No more than a few molecules of paired RNAs per cell were needed to obliterate a gene's expression. Moreover, the suppression of the gene could sometimes persist into subsequent generations of the roundworms. These observations dovetailed with peculiar results that other scientists had reported previously while experimenting on petunias and tobacco plants with antisense RNA and multiple copies of genes.
Mello and Fire realized that a novel gene-expression mechanism involving RNA could explain what was happening. They called it RNA interference and described it in a groundbreaking paper in 1998.
What they discovered was that when paired strands of RNA 20 to 30 nucleotides long are injected into a cell, a series of reactions peels them apart and links them to specialized enzymes called argonautes (or AGOs). The argonautes use these RNA templates to check for complementary mRNAs in a cell millions of times per second. When an argonaute finds close matches, it can destroy those mRNAs or enlist other proteins to block their translation, thus interfering with a gene's expression. This mechanism is so powerful and efficient at locating targeted RNAs that researchers in the field frequently liken it to a genetic "search engine."
RNAi appears to have evolved at least a billion years ago. It may have first arisen to help subdue viral infections, because the double-stranded RNA construct bears similarities to bits of viral genome. But whatever its original function, RNAi now operates in cells as part of a regulatory network that silences specific genes in ways that go beyond the traditional controls on transcribing and translating DNA. Indeed what makes the idea of RNAi so revolutionary is that it shows that the transcription of a gene is not just for the purpose of expressing a gene: transcription can be part of a feedback loop for regulating the gene, too.
One advantage of RNAi for cells is that it hugely increases the efficiency with which they can fine-tune the expression of their genes. For example, if a cell's environment abruptly changes, the cell may be stuck with leftover, untranslated mRNAs that in theory would commit it to a response that is no longer useful. RNAi can purge the cell of those obsolete mRNAs almost instantly and hasten the cell's adaptation to its current situation.
The more astonishing effect, however, is that RNAi's regulatory effects can pass from one generation to the next: offspring can inherit some of their parents' adaptations to an environment without a genetic change. This phenomenon of epigenetic inheritance has recently become the object of intense biomedical interest as researchers try to understand how passing environmental conditions may sometimes alter the physiology of people and other organisms throughout their lives and into later generations.
Now that the potency of RNAi at regulating genetic expression is known, the challenge is to turn it into a useful tool of biotechnology. As an experimental tool, it has already proved itself countless times in studies of basic gene function. But adapting it as a type of therapeutic drug compound, for instance, is complicated: because RNAi molecules are large and polarized, they do not easily or efficiently pass through the lipid membranes around cells, which prevents ones administered artificially from having their desired effects.
Mello has interests in developing RNAi applications, but much of his own research focus at present is on studying how RNAi and a menagerie of single-strand, non-coding RNA species naturally regulate genetic expression. (Much of his current work centers on tiny single-strand snippets called piwi RNA.) And as in the earlier stages of his work, C. elegans remains an indispensible partner as the research subject of choice. Studies in 2012 by Mello's group, for example, showed that the reproductive cells of C. elegans use complexes of short single-strand RNAs and argonaute proteins to scan their genome and silence the expression of parasitic sequences from viruses.
Mello moved from roundworms to gypsy moths for a 2014 study of the importance of RNAi to the insect's immunological defenses. Normally, certain viruses that can infect a wide variety of insect hosts have little success in attacking gypsy moths. Mello and his colleagues learned that the moths' resistance springs from natural RNAi molecules in their cells that block the replication of the viruses. But then the researchers also artificially infected the moth cells with vaccinia, the virus best known for its role in the development of a smallpox vaccine. Vaccinia disrupted the moths' protection against the other viruses, possibly by interfering with the RNAi mechanism's ability to target viral proteins for destruction.
That spirit of discovery that motivated Mello to wander through forests and dig for fossils when he was a boy has never faded. For him, the goal of understanding nature remains the noblest pursuit conceivable. As he noted in his Nobel autobiography, "The world is a far more remarkable place than we can imagine. Its mysteries define the human condition; to exist without knowing why." And as for himself? "I haven't changed much really in the intervening years. I'm still turning over stones, hoping to find something new."
© Scientific American 201619:T3cac,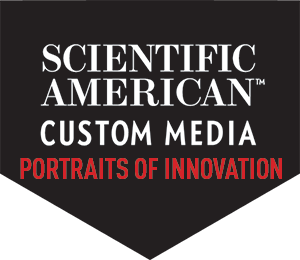
By John Rennie, Science Editor & Journalist
When a bite of spicy chili pepper seems to set your mouth aflame, the comparison is not just a figment of your imagination. Rather, the sensations of extreme spiciness and burning heat are intertwined in your nervous system at a molecular level: the same pain sensors scream out in response to both stimuli. Similarly, the refreshing coolness of menthol comes from your body’s impression that it is experiencing actual cold.
Those quirks of physiology embody important lessons about how evolution works. They could also be the key to developing more effective painkillers that would snuff out discomfort without causing the dangerous sleepiness of many current analgesics.
Credit for these surprising discoveries—and others—belongs to molecular physiologist David Julius, currently the chair of the physiology department at the School of Medicine of the University of California, San Francisco (UCSF). The string of remarkable finds emerging from his laboratory over the years has brought him professional acclaim and major awards including the first Perl-UNC Neuroscience Prize in 2000, the Run Run Shaw Prize in 2010, and more recently, the Dr. Paul Janssen Award for Biomedical Research in 2014.
Growing up as the middle son of three in Brighton Beach, a Russian-immigrant neighborhood of Brooklyn, N.Y., Julius had few thoughts of becoming a scientist. He spent one year as a student at the exclusive Stuyvesant High School in Manhattan, but then opted to transfer to the public high school closer to his home, having grown tired of incessant testing and grade competition at Stuyvesant. At Lincoln High, the tutelage of an entertaining physics teacher who showed his students the relevance of science to daily life made Julius reconsider his ambitions. He headed to the Massachusetts Institute of Technology in 1973 with plans to become a physician.
At M.I.T., however, the opportunities for hand-on research experience won him over. While a junior, Julius began bench work under Alexander Rich, a living legend in the study of biological macromolecules. “Alex’s lab was a creative, freewheeling, and messy place, dispelling any ideas I might have had about labs being stark and sterile environments reserved for quiet personalities and dispassionate experimentation,” Julius wrote of that experience in his Shaw Prize autobiography.
In 1977, Julius moved on to graduate studies in the biochemistry department of the University of California, Berkeley. His advisers were the noted biochemists Jeremy Thorner and Randy Schekman (who would win a Nobel Prize in 2013). His dual mentors gave him a rich appreciation of what the combined forces of molecular genetics and pharmacological biochemistry could do to probe cells’ inner workings. He set about studying yeast and the molecular mechanisms that regulate their production of peptide hormones.
Notwithstanding the enjoyable success Julius was having in studies of yeast molecular biology, he had also become intrigued about neurobiology in more complex organisms. He was especially intrigued about the possibility of using molecular genetics and pharmacological methods to decipher how neurons functioned. In 1984 he therefore pursued post-doctoral work at Columbia University with the neurobiologist Richard Axel (who, like Schekman, would also eventually be a Nobel laureate, in 2004).
“When I was there, everybody worked on something different,” Julius says. “The unifying theme was that everybody was trying to clone genes for key molecules” governing cell physiology. He set out to clone a serotonin receptor from rat brain, knowing that it would illuminate the role of serotonin in certain psychiatric conditions. Eventually, he succeeded in cloning the 5HT1c serotonin receptor.
As Julius’s focus of attention shifted to the nervous system, he became increasingly curious about why naturally occurring compounds in foods and other products could sometimes act on receptors in the brain and induce hallucinations or other changes in brain function. The conceptual gulf between receptor molecules in the brain and complicated brain phenomena seemed dauntingly vast. Still, he drew inspiration from eminent scientists like Solomon Snyder of Johns Hopkins Medical School, who had discovered how opioid compounds made by plants (like morphine) trigger receptors in the brain that respond to endogenous opioids.
When Julius moved on to UCSF to start his own lab in 1989, his first project was to clone another serotonin receptor of particular interest, one for a serotonin subtype called 5HT3. Julius and his group showed that neurons immediately fire when serotonin binds to 5HT3 receptor because the receptor, which is a gated ion channel, instantly opens. (In contrast, many serotonin receptors work more slowly and indirectly by releasing secondary messengers that in turn open ion channels.) That speed and efficiency made the 5HT3 receptor an appealing target for drug development.
PAIN WAS—AND IN MANY WAYS, STILL IS—A GREAT MYSTERY.
But Julius’s team also noticed that the 5HT3 receptor is expressed heavily in sensory neurons that are linked to the perception of pain (such as those in the dorsal root ganglia). That fact opened up an exciting new avenue for further research because pain was—and in many ways, still is—a great mystery.Neuroscientists had broken the phenomenon of pain into two components: nociception, the sensory process that detects noxious stimuli and signals to the central nervous system about them, and pain perception, which is the interpretive process that renders the sensory information into an unpleasant experience. Perception is complex because it integrates so many aspects of an individual’s condition and history (which is why anesthesiologists struggle to meaningfully quantify how much patients suffer).
Yet even nociception was murky in its details. At the time when Julius cloned the 5HT3 receptor, neuroscientists were divided about whether nociceptors even existed as a distinct class of sensory neurons: an opposing theory held that the brain and spinal cord recognized the signature of painful stimuli from some pattern or intensity in the neural signals for other touch sensations.
For decades, researchers had been studying pain in the laboratory with doses of capsaicin—the pungently spicy extract of chili peppers that gives them their kick. No one knew why capsaicin had this effect, however.
Julius hesitated to wade into such an unexplored area. Yet one day when he and his spouse, fellow UCSF professor Holly A. Ingraham, were at the supermarket, she found him staring at the spice aisle, lost in a reverie. She told him to take the plunge, and he did.
His lab’s initial efforts to find and clone the gene for the receptor that responded to capsaicin repeatedly failed because the gene was not active enough to be caught by conventional assays. The breakthrough came when Julius’s colleague Michael J. Caterina brought in new equipment that would allow them to map the influx of calcium ions into cells, a telltale sign of activity in neurons responsive to the capsaicin. Within about a month, they had cloned and isolated the gene for the capsaicin receptor, which they dubbed TRPV1. The TRPV1 receptor is a gated cation channel protein: like the 5HT3 receptor, it is a regulated pore in the cell membrane that can be triggered to let positive ions into a neuron.
The key question then was, what did this receptor do to signal pain in daily life when capsaicin was not around? Julius and his colleagues began throwing various other pain-inducing compounds at the receptor to little effect. The result was more dramatic, however, when they exposed the receptor to heat: at temperatures above 43°C (109°F), the receptor readily opened. This temperature also happens to be roughly the threshold for what the body normally considers an uncomfortable extreme of heat.
Julius, Caterina, and their colleagues published their discovery of TRPV1 in 1997 to huge acclaim. In the nearly two decades since that time, the laboratory has continued to study the behavior of the TRPV1 receptor, but also identified other receptors in the same molecular family that confirmed their status as painful temperature sensors. In 2002, Julius and Ardem Patapoutian of the Scripps Research Institute independently identified TRPM8, the receptor that conveys the cool sensation of menthol. Diana M. Bautista in Julius’s laboratory showed in 2007 that TRPM8 serves as a primary detector of environmental cold: it activates when temperatures drop below 26°C (79°F). In 2006, Sven-Eric Jordt in Julius’s group found that TRPA1 is the receptor that responds to the noxious irritation of wasabi, horseradish, and mustard, which differs from the spiciness of capsaicin. TRPA1 seems to help us sense irritating chemicals in the air, as well as compounds associated with inflammation.
The picture emerging from the work in Julius’s lab and elsewhere thus confirmed that nociceptors do exist as a distinct class of sensory neurons. Equally important was that it clarified how those pain receptors work. It even explained why the same receptors respond to stimuli as different as heat and chili extract.
When burning temperatures trigger the TRPV1 receptors, for example, the body immediately reacts to the signal by mounting an inflammatory response around the injured area. Some of the natural substances released in that response also interact with TRPV1 receptors and make them more responsive. Think of how your skin feels immediately after a sunburn: it becomes uncomfortably sensitive to even small amounts of heat.
On the other hand, the capacity of those nociceptors to respond is not inexhaustible. That could be why topical pain-relieving ointments containing capsaicin bring relief: their gentle, prolonged stimulation of the TRPV1 receptors may desensitize the neurons and reduce the local inflammatory response.
For drug developers, the fact that specific components of pain sensation can be teased apart at a molecular level is exciting. It should be possible to develop analgesics that can selectively subdue the pain of inflammation, for example, without the broad side effects of opioid painkillers. Several experimental painkillers aimed at TRPV1 receptors have gone into clinical trials, but an initial problem has been that they had the undesirable effect of causing fevers or diminishing the protective response to heat.
According to Julius, these effects may be an “on-target effect,” an incidental consequence of diminishing sensitivity to noxious heat. “Because those sensors are also involved in detecting environmental temperature, you thermoadapt,” he says. “That’s why people like to eat hot peppers in hot climates.” The hope, he says, is that future drugs targeting TRPV1 or TRPA1 may block the receptor’s modulation of sensations more selectively. Rather than blocking the receptor outright, he says, those drugs would subtly modulate its behavior, taking out the hypersensitivity to pain from inflammation but leaving the environmental sensitivity important for maintaining healthy body temperatures.
Pharmaceutical applications aside, however, for Julius, learning how sensory receptor molecules work in more detail is reward enough. His work also lets him appreciate the inventiveness of evolution in finding radically novel uses for established molecules.
Chili peppers evolved capsaicin, for example, because it served as a defensive exploitation of animals’ inflammatory pain mechanism. Hungry creatures that ate those plants paid a price in fiery discomfort. The capsaicin-triggered pain receptor is a vulnerability that nature has exploited on other occasions, too: in 2006 Julius’s lab discovered that it is also the key to why the venom in a tarantula’s bite is devastatingly painful.
“We’ve enjoyed looking at sensory systems from that perspective,” Julius says. One night when he and his family were watching a television documentary about rattlesnakes, he idly wondered whether the snakes’ ability to sense the body heat had anything to do with the receptor molecules he was studying. At that time, researchers still debated whether snakes’ infrared-sensitive pit organ might have evolved out of the visual system.…FINDING THE RIGHT BALANCE BETWEEN CHASING EXCITING IDEAS THAT MIGHT TRANSFORM FIELDS AND KEEPING A MORE SUSTAINED FOCUS THAT YIELDS DETAILED KNOWLEDGE AND DEFINES YOUR EXPERTISE.
In 2010, Elena O. Gracheva, Julius, and other members of his lab published proof that the infrared detector in the pit organ was an adaptation of the wasabi-sensitive TRPA1 receptor—a noxious chemical sensor co-opted to work as a heat sensor. The following year, they showed that vampire bats find vulnerable blood vessels in their warm-blooded prey with the help of heat sensors around the nose derived from TRPV1.Julius thinks that sensory systems are a particularly fruitful place to look for molecular adaptations. Whereas the molecules that handle basic housekeeping chores for the nervous system tend to be similar among all animals, he says, “in sensory systems you see more rapid evolution of molecules as they respond to the salient environment of that organism.” Toxin biology is another great area for such evolution studies, he adds. “Animals have had millions of years to evolve toxins. Molecules get repurposed over and over again.”Reflecting on the diverse successes of his career, Julius notes that “attracting great people to your lab” and having reliable funding for curiosity-based research are always key. In his own studies, he credits finding the right balance between chasing exciting ideas that might transform fields and keeping a more sustained focus that yields detailed knowledge and defines your expertise. “There comes a point when you find the things you’re really interested in,” Julius says, “and then you do deep.”
READINGS
Bautista DM et al. (2006) TRPA1 mediates the inflammatory action of environmental irritants and proalgesic agents. Cell 124: 1269-1282. doi: 10.1016/j.cell.2006.02.023
Bautista DM. (2015) Spicy science: David Julius and the discovery of temperature-sensitive TRP channels. Temperature. 2(2): 135-141. doi: 10.1080/23328940.2015.1047077
Caterina MJ et al. (1997) The capsaicin receptor: a heat-activated ion channel in the pain pathway. Nature 389:816-824. doi:10.1038/39807
Clapham DE. (1997) Some like it hot: spicing up ion channels. Nature 389:783-784. doi:10.1038/39724
Grachevna EO et al. (2010) Molecular basis of infrared detection by snakes. Nature. 464:1006-1012. doi:10.1038/nature08943
Grachevna EO et al. (2011) Ganglion-specific splicing of TRPV1 underlies infrared sensation in vampire bats. Nature. 476:88-92. doi: 10.1038/nature10245
Julius D. (2010) Autobiography of David Julius. The Shaw Prize. Web. 2010 Sept 28.1a:T3687,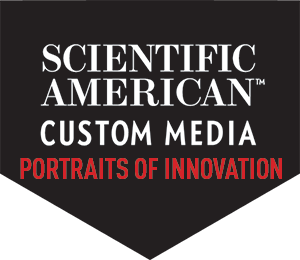
By John Rennie, Science Editor & Journalist
When four-year-old Melissa’s parents brought her into the emergency room of Johns Hopkins Hospital one Friday night in 1974, it fell to young Dr. Bert Vogelstein, the new resident in charge, to tell them the diagnosis was leukemia. Her parents absorbed the news as best they could, hoping for a cure that didn’t exist, but they also desperately needed an answer: why was she sick? Had they done something wrong? Something they should have done differently to prevent the cancer?
Vogelstein tried to reassure them they were not to blame, but he knew the heartbreaking truth was that medical science had no answer. “I was just being polite,” he recalls. “I had absolutely no idea of what caused this little girl’s illness” because cancer was a “total black box.”
That nagging, crucial question—what caused this cancer?—was one Vogelstein heard over and over again from parents, children, and others during his two-year pediatrics residency. His frustration over it, Vogelstein says, is what propelled him into the laboratory. “You’ve got to try and change that,” he recalls thinking. “Whether you can or not, we don’t know, but at least you’ve got to try.” It initiated the decades of astonishing research success studded with genuine scientific breakthroughs that have defined Vogelstein’s career.
VOGELSTEIN IS CREDITED WITH DEVELOPING THE DETAILED, GENE-CENTERED UNDERSTANDING THAT MODERN SCIENCE HAS ABOUT HOW ALL CANCERS ARISE…
For example, Vogelstein and his collaborators were the first to explain the precise genetic process by which a common human malignancy, colorectal cancer, originates in healthy tissues. More broadly, Vogelstein is credited with developing the detailed, gene-centered understanding that modern science has about how all cancers arise: he showed that they are diseases that evolve through generations of cells and the gradual accumulation of specific mutations that make them increasingly lethal to their host. His research group is credited with identifying the genetic basis of at least 10 types of cancer, more than any other laboratory. The phenomena that Vogelstein identified have become the foundations of new anti-cancer therapies, diagnostics, and preventative strategies. Because of the importance of his work and his prolific authorship of more than 450 papers, Vogelstein is the most cited scientist in the world—not just in cancer research or even in biology, but in all of science. In recognition of this amazingly rich record of accomplishment, Vogelstein was named as the 2015 winner of the Dr. Paul Janssen Award for Biomedical Research honors.
A Baltimore native born in 1949, Vogelstein was an enthusiastic independent reader as a boy, although he frequently skipped out of classes at the private middle school he attended. He majored in mathematics as an undergraduate at the University of Pennsylvania and graduated summa cum laude in 1970. For a brief time he worked toward a master’s degree in mathematics, but he eventually decided that medicine and human health fascinated him more, so he instead pursued a medical degree from the Johns Hopkins University School of Medicine. After graduating in 1974 and serving his formative pediatric residency at Johns Hopkins Hospital, Vogelstein worked as a research associate of the National Cancer Institute between 1976 and 1978, until Johns Hopkins University appointed him as an assistant professor of oncology.
As an oncology researcher, Vogelstein could finally begin to explore how the origins of cancer might be genetic. Today, the idea that cancer results from genes gone wrong is practically common knowledge. Yet it was not always so: by the 1970s scientists had begun to implicate a variety of genes in cancer but there was still little consensus about whether the blame for triggering a tumor’s growth and spread lay primarily with mutations, failures of the immune system, or infectious agents like viruses. Vogelstein was most suspicious of mutations, but proving that theory—or even investigating it—had stymied most efforts.
He and his team began to look at the progression of human colorectal tumors at a level of genetic detail. (Colorectal tumors were their subject of choice because obtaining sequential tissue samples from patients over time was relatively easy.) A particular innovation they brought to their approach was to study how individual clones of tumor cells changed and proliferated inside the body. They accomplished this by restriction fragment length polymorphism (RFLP) analysis, a DNA profiling technique that can identify telltale differences in the base sequences of corresponding genes in different cells.
Vogelstein’s group found that in more than half the tumors they studied, the cells had mutations in one particular gene called K-ras. That discovery made sense in that K-ras had previously been classified as an oncogene, a gene that in its normal form signaled for cells to grow and that when mutated signaled them to grow uncontrollably. But a single mutation could not by itself be causing cancer. Rather, as Vogelstein proposed in 1988, cancer more likely the result of mutations in several specific classes of genes, including oncogenes, that accumulated sequentially. As clones of tumor cells replicated, they acquired more mutations over generations and became increasingly deranged and unruly, until some of them bloomed into full malignancies.
Vogelstein confirmed that hypothesis in 1989, the year in which he was named a professor of oncology at Johns Hopkins, when he and his colleagues published a seminal paper on mutations in the p53 gene on chromosome 17. They showed that p53 was mutated not just in most colon cancers but also in more than half of all cancerous tumors. (Indeed, subsequent work has confirmed that p53 is the most commonly mutated gene in cancers.) It had been known that p53 was involved in cancer for 10 years, which is why it had been classified as an oncogene. But Vogelstein’s work showed conclusively that p53 instead belonged to a different class: it was a tumor-suppressor gene, one that normally suppressed replication in abnormal cells. Thus, mutations in p53 cut the brakes on a process that should stop new tumors from expanding.
Vogelstein’s lab followed the p53 paper with studies that identified more tumor-suppressor genes, including one they called APC in 1991. (The APC gene was also discovered independently at the same time by Ray White and his laboratory at the University of Utah.) APC is mutated in the inherited condition called familial adenomatous polyposis, in which patients develop huge numbers of benign tumors. Vogelstein and Kenneth W. Kinzler, who was then a graduate student but has since become Vogelstein’s longtime laboratory colleague and a full professor at Johns Hopkins, showed that APC is also the gene that first spontaneously mutates in most cases of colorectal cancer. If a cell lining the colon gets an APC mutation, it spawns abundant benign tumors, or adenomas, in which further mutations and malignancy can emerge.
Further work by Vogelstein and his colleagues showed that mutations in the genes that enable the DNA repair mechanism called mismatch repair (or MMR) also help to enable tumorigenesis and cancer. When strands of DNA are replicating, MMR proofreads the new strands to ensure that inappropriate nucleotide bases are cut out and replaced with the right ones. Defects in MMR lead directly to more mutations and faster progression toward cancer.
Thanks to Vogelstein and his team, oncologists now know that cancers arise through mutations acquired in three classes of genes—oncogenes, tumor-suppressor genes, and DNA repair genes—which betters understanding both of how tumors progress and of why cancers can be so diverse and persistent. The challenge for modern oncology is in putting those insights to work in new diagnostics, treatments, and preventatives. It has already born fruit, and Vogelstein has continued to contribute powerfully to those efforts.
For example, back in 1999, Vogelstein and Kinzler described digital PCR, a technique that improved on the more traditional quantitative polymerase chain reaction-based method for finding specific mutations in complex DNA samples. They upgraded it dramatically in 2003 with a further procedure called BEAMing (for beads, emulsion, amplification, and magnetics) that used individual magnetic beads as anchors for thousands of identical DNA fragments to make digital PCR much easier and faster.
For example, back in 1999, Vogelstein and Kinzler described digital PCR, a technique that improved on the more traditional quantitative polymerase chain reaction-based method for finding specific mutations in complex DNA samples. They upgraded it dramatically in 2003 with a further procedure called BEAMing (for beads, emulsion, amplification, and magnetics) that used individual magnetic beads as anchors for thousands of identical DNA fragments to make digital PCR much easier and faster.
Digital PCR and BEAMing are now routinely used for genome analysis by laboratories everywhere. And Vogelstein and Kinzler put them to use, starting in 2004, in a massive project aimed at studying the more than 150 protein-making genes in human DNA involved in the development of tumors. The tumor-linked sequences they found are useful as biomarkers for monitoring the progression of tumors in patients.
Vogelstein has also worked on novel treatments for cancer, such as the use of bacteria to fight tumors. As solid tumors grow, their cores often become oxygen-starved because of their poor blood supply. To take advantage of that weakness, his group experimented with injecting modified versions of soil bacteria (Clostridium novyi) into tumorous laboratory animals. The bacteria, which thrive under low oxygen conditions, colonized the tumors and multiplied but stayed out of healthy tissues. Toxins released by the bacteria directly killed the surrounding tumor cells. The bacterial treatment, which is being co-developed by Johns Hopkins and BioMed Valley Discoveries, is now undergoing further animal and clinical study.
Encouraging as such treatment possibilities might be, Vogelstein nevertheless believes that the best and most realistic opportunities for fighting cancer are in prevention, early diagnosis, and early treatment rather than in cures for advanced forms of the disease. For him, that view follows logically from the recognition that cancers arise through a process of gradual transformation: it takes on average almost 30 years for cells nudged into becoming tumors by an APC mutation to acquire all the other mutations essential to colon cancers. That’s three decades in which cells drifting toward malignancy could potentially be found and stopped.
“Of course, we have to continue to cure advanced disease because no prevention net will capture all of them,” Vogelstein remarked on-stage during the Dr. Paul Janssen Award celebrations in his honor. “But unless we use all of the weapons at our disposal including early detection and prevention, my fear is that 50 years from now we’ll still be trying to mainly cure advanced cancers, which is unacceptable.”
Indeed, although Vogelstein staunchly supports trying to curb people’s exposures to carcinogenic influences in the environment, he also recognizes that prevention has its limits. Early in 2015, Vogelstein and Cristian Tomasetti published a paper that became controversial for seeming to say that “bad luck” causes two thirds of all cancers. That flip summary does not do justice to their finding, however: Vogelstein and Tomasetti’s analysis concluded that most of the mutations causing cancers result from the random errors inherent in the mechanisms responsible for normal cell replication—not from any inherited or environmental factors. As such, a certain level of risk is inevitable over time, no matter how carefully one tries to prevent it.
In recent years, therefore, Vogelstein interest in chasing new cancer genes has faded in favor of identifying telltale signs of cancers earlier. He and his colleagues have been pioneers in the development of a noninvasive “liquid biopsy” approach to cancer diagnosis. Tumor cells shed their DNA into the bloodstream, often in surprising abundance. Sensitive DNA assays of a patient’s blood sample should in theory not only be able to spot the signature of a growing tumor but even identify the tissue in which it resides. The technique is in clinical development by several groups, including Vogelstein’s, and has shown great promise in animal tests. Skeptics have raised concerns, however, about whether the ability to diagnose cancers without always being able to do anything about them will put patients and physicians in a difficult bind.
THINK LIKE A SCIENCE FICTION WRITER AND THEN USE YOUR SCIENTIFIC SKILLS TO REALIZE THAT FUTURE.
During his Dr. Paul Janssen Award celebrations, Vogelstein advised young investigators choosing directions for their career to think more like science fiction writers than like scientists. Scientists, he warned, can be “too tethered to reality” to recognize future possibilities. “Think like a science fiction writer and then use your scientific skills to realize that future.” He also suggested that young scientists imagine having succeeded in their goals and then writing to their mother about what they had done. “Would she be excited?” he asked. “Mothers are pretty discerning.”
© Scientific American 20160:["cQBEQHCL5b9I7J0ijoHz7",[[["",{"children":["newsroom",{"children":["__PAGE__",{}]}]},"$undefined","$undefined",true],["",{"children":["newsroom",{"children":["__PAGE__",{},[["$L1",["$","main",null,{"className":"bg-white","children":["$","div",null,{"className":"min-h-20 ","children":["$","$L2",null,{"data":[{"id":0,"title":"Biomedical scientist Robert Langer receives the 2023 Dr. Paul Janssen Award for Biomedical Research","image":"/images/RobertLanger.png","blogimageheight":600,"blogimagewidth":855,"blogimage":"/images/Blog_RobertLanger.png","date":"February 2023","videolink":"https://players.brightcove.net/3198958923001/9Z7fsGThCO_default/index.html?videoId=6346073082112","videolink2":"","question":"","summary":"$3"},{"id":1,"title":"A Science Award Unlike Any Other","image":"/images/Thumbnail-Champion of science.jpg","blogimageheight":600,"blogimagewidth":855,"blogimage":"/images/D6774933-EE2F-4355-920EA60E44DBAE9E_source.jpg","date":"February 2021","videolink":"","videolink2":"","question":"The Dr. Paul Janssen Award has anticipated profound transformations in biomedicine and more than a few Nobel Prizes. What makes it so coveted among researchers?","summary":"$4"},{"id":2,"title":"2020 DPJA Symposium e-briefing","image":"/images/CantleyInLabAdjusted.jpg","blogimageheight":600,"blogimagewidth":855,"blogimage":"/images/DrCantley.png","date":"January 2021","videolink":"","videolink2":"","question":"","summary":"Dr. Lewis Cantley received the 2020 Dr. Paul Janssen Award for his discovery of PI-3-kinase (PI3K). His groundbreaking research led to the development of a new class of drugs called phosphoinositide-3 kinase inhibitors, and has revolutionized treatment for cancer, diabetes and autoimmune diseases.
In September, Dr. Cantley’s work was celebrated with the 2020 Dr. Paul Janssen Award Symposium at the New York Academy of Sciences. Learn more in an e-briefing from the event, available here."},{"id":3,"title":"DPJA Alums Honored with Nobel Prize","image":"/images/DPJA-prize-blog.png","blogimageheight":720,"blogimagewidth":880,"blogimage":"/images/DPJA-prize-blog (1).png","date":"October 2020","videolink":"https://players.brightcove.net/3198958923001/9Z7fsGThCO_default/index.html?videoId=6198374110001","videolink2":"","question":"","summary":"Drs. Jennifer Doudna and Emmanuelle Charpentier, who won the Dr. Paul Janssen Award for Biomedical Research in 2014, have received the 2020 Nobel Prize in Chemistry. Johnson & Johnson was proud to recognize Doudna and Charpentier in 2014 for their discovery of the CRISPR-Cas9 method of genetic editing, considered one of the most significant breakthroughs in molecular biology in the past decade which has led to a substantial leap in scientists’ ability to manipulate genetic processes and holds promise for significantly advancing drug development."},{"id":4,"title":"2020 Dr. Paul Janssen Winner","image":"/images/Dr. Cantley 2020 DPJA.png","blogimageheight":720,"blogimagewidth":880,"blogimage":"/images/Dr. Cantley 2020 DPJA_newsroom.png","date":"October 2020","videolink":"https://players.brightcove.net/3198958923001/9Z7fsGThCO_default/index.html?videoId=6198446055001","videolink2":"","question":"","summary":"Lewis Cantley, Ph.D. of Weill Cornell Medicine, reflects on winning the 2020 Dr. Paul Janssen Award for Biomedical Research. A world leader in cancer and metabolic disease research, he was selected by an independent committee of renowned scientists for significant advances in cancer research for his discovery of PI-3-kinase (PI3K) which has revolutionized treatments for cancer, diabetes and autoimmune diseases.
Dr. Cantley joins 18 other researchers who've received the award to date, including five who were ultimately awarded a Nobel Prize. Watch the video."},{"id":5,"title":"2020 Dr. Paul Janssen Award Webcast","image":"/images/image.jpg","blogimageheight":720,"blogimagewidth":880,"blogimage":"/images/image_newsroom.jpg","date":"September 2020","videolink":"https://players.brightcove.net/3198958923001/9Z7fsGThCO_default/index.html?videoId=6193274559001","videolink2":"","question":"","summary":"The time to champion science is now.
Science impacts our daily lives in every imaginable way, fueling progress and delivering the next generation of treatments and cures. Now, more than ever, science needs champions... to help us understand how research and innovation can help create a better future.
Join us LIVE for the Dr Paul Janssen Award as we celebrate scientific heroes whose curiosity and tenacity are leading to longer, healthier, happier lives for people all over the world. As Dr Paul said, “There is so much more to be done; the patients are waiting.”
Champions of Science: The Dr Paul Janssen Award, Wednesday September 16th at 1 EST."},{"id":6,"title":"2020 Winner: Dr. Lewis C. Cantley","image":"/images/dr-lewis-blog-image.png","blogimageheight":700,"blogimagewidth":850,"blogimage":"/images/Dr. Lewis Cantley_Weill Cornell_019.jpg","date":"August 2020","videolink":"","videolink2":"","question":"","summary":"Lewis Cantley, Ph.D., has made significant contributions to cancer research stemming from his discovery of the signaling pathway phosphoinositide 3-kinase (PI3K) in 1984. A graduate of West Virginia Wesleyan College (B.S., Chemistry, 1971) and Cornell University (Ph.D., Biophysical Chemistry, 1975), Dr. Cantley has been a professor at Tufts University and Harvard University.
He served director of the Beth Israel Deaconess Cancer Center and is currently the Meyer Director of the Sandra and Edward Meyer Cancer Center at Weill Cornell Medicine. The author of more than 400 papers and 50 book chapters, Dr. Cantley has received several prestigious accolades, including membership in the American Academy of Arts and Sciences, the National Academy of Sciences, the Institute of Medicine of the National Academies, and the European life sciences academy EMBO."},{"id":7,"title":"Honoring 2019 Dr. Paul Janssen Award Winners, Drs. Hartl and Horwich","image":"/images/DrHorwichspeaking_0.jpg","blogimageheight":700,"blogimagewidth":850,"blogimage":"/images/DrHorwichspeaking_blog_0.jpg","date":"October 2019","videolink":"","videolink2":"","question":"2019 Dr. Paul Janssen Award winners, Drs. Hartl and Horwich were celebrated at events in Beerse – the home of Dr. Paul, and New York City. Following are remarks from Chair of the Selection Committee, David Julius, Ph.D. Dr. Julius is professor and chair of the Department of Physiology at the University of California, San Francisco, and was a winner of the Dr. Paul Janssen Award in 2013.","summary":"$5"},{"id":8,"title":"2019 Event Highlights","image":"/images/BeerseDPJA2019_27092019-8682_resize.jpg","blogimageheight":700,"blogimagewidth":850,"blogimage":"/images/BeerseDPJA2019_27092019-8682_resize_blog.jpg","date":"October 2019","videolink":"https://players.brightcove.net/3198958923001/default_default/index.html?videoId=6096539575001","videolink2":"","question":"","summary":"Dr. Franz Ulrich-Hartl, of the Max Planck Institute for Biochemistry and Dr. Arthur Horwich, of the Yale School of Medicine and Howard Hughes Institute are the recipients of the 2019 Dr. Paul Janssen Award for Biomedical Research. The duo is known for their revolutionary work in understanding of how chaperone-mediated protein folding contributes to human biology and health.
As part of our annual celebration, Dr. Hartl and Horwich were honored by the scientific community across two continents in Beerse, Belgium, the home of Dr. Paul and New York. Both events included scientific symposia. Watch the video to see highlights from this year’s events honoring the legacy of Dr. Paul Janssen."},{"id":9,"title":"2019 Dr. Paul Janssen Award Winners: Franz-Ulrich Hartl & Arthur Horwich","image":"/images/HartlHorwich_DPJA_website_images364 x 168.jpg","blogimageheight":700,"blogimagewidth":850,"blogimage":"/images/HartlHorwich_DPJA_website_image2_910 x 607_v1a.jpg","date":"June 2019","videolink":"https://players.brightcove.net/3198958923001/default_default/index.html?videoId=6042738045001","videolink2":"https://players.brightcove.net/3198958923001/default_default/index.html?videoId=6043244757001","question":"","summary":"$6"},{"id":10,"title":"2018 Event Highlights","image":"/images/dpja2018_belgium_0.jpg","blogimageheight":700,"blogimagewidth":850,"blogimage":"/images/dpja2018_belgium_full.jpg","date":"September 2018","videolink":"https://players.brightcove.net/1492317933001/default_default/index.html?videoId=5840407030001","videolink2":"","question":"","summary":"$7"},{"id":11,"title":"Behind the Revolution in Cancer Therapy","image":"/images/cancer therapy2.png","blogimageheight":700,"blogimagewidth":850,"blogimage":"/images/cancer therapy2_blog.png","date":"September 2018","videolink":"https://players.brightcove.net/1492317933001/default_default/index.html?videoId=5836372234001","videolink2":"","question":"","summary":"$8"},{"id":12,"title":"4 Questions for Immunotherapy Pioneer Dr. James Allison","image":"/images/DPJA_James_Allison_interview.jpg","blogimageheight":700,"blogimagewidth":850,"blogimage":"/images/DPJA_James_Allison_winner_homepage3.jpeg","date":"September 2018","videolink":"","videolink2":"","question":"","summary":"$9"},{"id":13,"title":"A survivor’s story","image":"/images/video_Sharon_Belvin_3_video_thumb.png","blogimageheight":700,"blogimagewidth":850,"blogimage":"/images/video_Sharon_Belvin.png","date":"September 2018","videolink":"https://players.brightcove.net/1492317933001/default_default/index.html?videoId=5831140430001","videolink2":"","question":"","summary":"Sharon Belvin was a 22 year old grad student just two weeks away from her wedding when she received news that would forever change her life. Sharon was diagnosed with late stage melanoma. Every treatment available had failed her, until she joined a clinical trial in 2005 for an immunotherapy developed by the 2018 Dr. Paul Janssen Award winner, Dr. James Allison. Hear Sharon’s incredible story here."},{"id":14,"title":"2018 Dr. Paul Janssen Award Winner; Dr. James Allison!","image":"/images/1800x834_ DrJamesAllison_video_new.jpg","blogimageheight":700,"blogimagewidth":850,"blogimage":"/images/video_screenshot_blog.png","date":"June 2018","videolink":"https://players.brightcove.net/1492317933001/default_default/index.html?videoId=5792518125001","videolink2":"","question":"","summary":"$a"},{"id":15,"title":"Video Highlights: 2017 Dr. Paul Janssen Award Celebration Honoring Doug Wallace","image":"/images/TJK_0801_play.jpg","blogimageheight":700,"blogimagewidth":850,"blogimage":"/images/TJK_0801-1301x603.jpg","date":"September 2017","videolink":"https://players.brightcove.net/1492317933001/default_default/index.html?videoId=5587981840001","videolink2":"","question":"","summary":"Dr. Douglas Wallace of the Children’s Hospital of Philadelphia is the recipient of the 2017 Dr. Paul Janssen Award for Biomedical Research for his pioneering work in the field of mitochondrial genetics.
Dr. Wallace’s work has led to a treasure trove of insights into our genealogy, and has implications for understanding and treating a range of metabolic and degenerative diseases, cancer and aging. Dr. Wallace overcame many obstacles during his 47-year career journey, and is a true Champion of Science who emulates the tenacity and legacy of Johnson & Johnson’s own trailblazer, Dr. Paul Janssen.
The scientific community came together to celebrate Dr. Wallace during recent events in New York and Beerse, Belgium -- the home of Dr. Paul. Watch the video to see highlights from this year’s events!"},{"id":16,"title":"CHOP Colleagues Congratulate Dr. Wallace on the 2017 Dr. Paul Janssen Award","image":"/images/1301x603_DrTaylor.jpg","blogimageheight":700,"blogimagewidth":850,"blogimage":"/images/1301x603_DrTaylor_noplay.jpg","date":"September 2017","videolink":"https://players.brightcove.net/1492317933001/default_default/index.html?videoId=5572122755001","videolink2":"","question":"","summary":"Hear from colleagues of the 2017 Dr. Paul Janssen Award recipient, Douglas Wallace, Ph.D., as they offer their warm congratulations and reflect on the importance of Dr. Wallace’s research and contribution to the field of mitochondrial genetics."},{"id":17,"title":"Everyone can be a Champion of Science!","image":"/images/1301x603_Meredith.jpg","blogimageheight":700,"blogimagewidth":850,"blogimage":"/images/1301x603_Meredith_noplay.jpg","date":"September 2017","videolink":"https://players.brightcove.net/1492317933001/default_default/index.html?videoId=5572273859001","videolink2":"","question":"","summary":"Meredith Hardy is a mom whose journey with Mitochondrial disease has turned her into a true Champion of Science. Meredith’s sons were both diagnosed with Mitochondrial disease over a decade ago and have been dealing with it’s severe, life threatening symptoms ever since. Hear how the work of 2017 Dr. Paul Janssen Award recipient, Dr. Douglas Wallace, has had an impact on Meredith’s family and the Mito community, who are waiting for new solutions."},{"id":18,"title":"VICTOR AMBROS, PH.D. AND GARY RUVKUN, PH.D.","image":"/images/POI-Ambros-Ruvkun_1.jpg","blogimageheight":700,"blogimagewidth":850,"blogimage":"/images/POI-Ambros-Ruvkun.jpg","date":"August 2017","videolink":"","videolink2":"","question":"","summary":"$b"},{"id":19,"title":"Podcast: Download & listen!","image":"/images/Seema Blog Post_1304x603.jpg","blogimageheight":700,"blogimagewidth":850,"blogimage":"/images/Seema Blog Post_1304x603_blog.jpg","date":"August 2017","videolink":"https://www.podbean.com/player-v2/?from=old_player&btn-skin=7&mini=0&rtl=0&fonts=Helvetica&download=1&share=1&start_at=0&i=h5zqm-6c274f-pb","videolink2":"","question":"","summary":"By Seema Kumar
08/2018
Why does science need champions?
Why is it important to celebrate and champion science? Listen to Seema Kumar, Vice President of Innovation, Global Health and Policy Communication at Johnson & Johnson, share her perspective about why now, more than ever, science needs champions.
Use and follow #ChampionsofScience to show your support. For every use of #ChampionsofScience, Johnson & Johnson will donate $5, up to $50,000, to the Biotechnology Institute through September 30 to engage and empower the next generation of scientific innovators ."},{"id":20,"title":"The Maternal Powerhouse","image":"/images/Carol Robinson Blog Post 1304x603.jpg","blogimageheight":700,"blogimagewidth":850,"blogimage":"/images/Carol Robinson Blog Post 1304x603_blog.jpg","date":"June 2017","videolink":"","videolink2":"","question":"","summary":"$c"},{"id":21,"title":"2017 Dr. Paul Janssen Award Winner; A True Champion of Science","image":"/images/CompilationVideo-Image-1301x603_0_playbtn3.jpg","blogimageheight":700,"blogimagewidth":850,"blogimage":"/images/CompilationVideo-Image-1301x603_0.jpg","date":"June 2017","videolink":"https://players.brightcove.net/1492317933001/default_default/index.html?videoId=5474224436001","videolink2":"","question":"","summary":"Now, more than ever, science needs champions. Johnson & Johnson is proud to be a champion of science and recognize the work of researchers through programs like the Dr. Paul Janssen Award for Biomedical Research. This year’s winner is Douglas Wallace, Ph.D. Founder and Director, Center for Mitochondrial and Epigenomic Medicine, Children’s Hospital of Philadelphia. Dr. Wallace’s pioneering work in the field of mitochondrial genetics -- and its application to the study of disease, aging, and patterns of human migration – has been groundbreaking. Hear from Dr. Wallace, Seema Kumar, Vice President of Innovation, Global Health and Policy Communication, Johnson & Johnson and Paul Stoffels, M.D., Executive Vice President, Chief Scientific Officer as they reflect on the importance of this award and the prolific legacy of Dr. Paul Janssen himself."},{"id":22,"title":"Join us in Championing Science","image":"/images/SizzleReel-Image-1301x603_OPT3_playbtn3.jpg","blogimageheight":700,"blogimagewidth":850,"blogimage":"/images/SizzleReel-Image-1301x603_OPT3_0.jpg","date":"June 2017","videolink":"https://players.brightcove.net/1492317933001/default_default/index.html?videoId=5476642731001","videolink2":"","question":"","summary":"Science is everywhere. It impacts our lives in every imaginable way. Science is knowing… caring… opportunity… hope. To continue to progress, science needs champions.
In celebration of the contributions of dedicated researchers around the world, and to fuel the next generation of innovators, Johnson & Johnson will donate $5, up to $50,000, to the Biotechnology Institute every time someone shows support for science by using #ChampionsofScience
Tell the world why you are a champion of science and inspire others to show their support!"},{"id":23,"title":"Be a Champion of Science","image":"/images/SeemaBlogPost-1301x603.jpg","blogimageheight":700,"blogimagewidth":850,"blogimage":"/images/SeemaBlogPost-1301x603_blog.jpg","date":"June 2017","videolink":"","videolink2":"","question":"","summary":"$d"},{"id":24,"title":"Emmanuelle Charpentier, Ph.D. and Jennifer Doudna, Ph.D.","image":"/images/Drs. Doudna and Charpentier - No logo_0.jpg","blogimageheight":700,"blogimagewidth":850,"blogimage":"/images/Drs. Doudna and Charpentier - No logo_0_blog.jpg","date":"March 2017","videolink":"","videolink2":"","question":"","summary":"$e"},{"id":25,"title":"Do You Know the Next Dr. Paul Janssen Award Winner?","image":"/images/DPJA_Medallion_1301x603b.jpg","blogimageheight":700,"blogimagewidth":850,"blogimage":"/images/DPJA_Medallion_1301x603b_blog.jpg","date":"January 2017","videolink":"","videolink2":"","question":"","summary":"$f"},{"id":26,"title":"Highlights from the 2016 Dr. Paul Janssen Award Celebration","image":"/images/Paul Janssen Award Highlights Post_playbtn3.jpg","blogimageheight":700,"blogimagewidth":850,"blogimage":"/images/Paul Janssen Award Highlights Post.jpg","date":"October 2016","videolink":"https://players.brightcove.net/3198958923001/default_default/index.html?directedMigration=true&videoId=5153504898001&","videolink2":"","question":"","summary":"This year's Dr. Paul Janssen Award for Biomedical Research was awarded to Dr. Yoshinori Ohsumi of the Tokyo Institute of Technology. Dr. Ohsumi was honored for his pioneering discoveries concerning the molecular basis of autophagy—a basic function of all living cells—which holds promise for better understanding, diagnosing and treating many diseases. Johnson & Johnson executives and members of the scientific community celebrated Dr. Ohsumi for his groundbreaking work during recent events in New York and Beerse, Belgium, demonstrating J&J's commitment to celebrating scientists and scientific discovery. The Dr. Paul Janssen Award honors the enduring legacy of Dr. Paul and recognizes the power of innovation to make a difference in human health."},{"id":27,"title":"CELEBRATING THE POWER OF SCIENCE","image":"/images/PaulStoffels-1601x603.jpg","blogimageheight":700,"blogimagewidth":850,"blogimage":"/images/PaulStoffels-1601x603_blog.jpg","date":"October 2016","videolink":"","videolink2":"","question":"","summary":"$10"},{"id":28,"title":"Where can fundamental research in biology lead us?","image":"/images/the_power_of_basic_science.jpg","blogimageheight":700,"blogimagewidth":850,"blogimage":"/images/the_power_of_basic_science_.jpg","date":"September 2016","videolink":"","videolink2":"","question":"","summary":"$11"},{"id":29,"title":"Of yeast and men. How to survive starvation and infectious disease.","image":"/images/BlogImage_Yeast_Men.jpg","blogimageheight":700,"blogimagewidth":850,"blogimage":"/images/BlogImage_Yeast_Men_blog.jpg","date":"June 2016","videolink":"","videolink2":"","question":"","summary":"$12"},{"id":30,"title":"Paul Janssen","image":"/images/DrPaulJanssen_PortraitofInnovation1.jpg","blogimageheight":700,"blogimagewidth":850,"blogimage":"/images/DrPaulJanssen_PortraitofInnovation1_1.jpg","date":"May 2016","videolink":"","videolink2":"","question":"","summary":"$13"},{"id":31,"title":"The Difference between Interesting and Useful","image":"/images/Bert-Video-Blog_playbtn3.jpg","blogimageheight":700,"blogimagewidth":850,"blogimage":"/images/Bert-Video-Blog.png","date":"May 2016","videolink":"https://players.brightcove.net/3198958923001/default_default/index.html?directedMigration=true&videoId=4499778193001&","videolink2":"","question":"","summary":"Dr. Bert Vogelstein, 2015 Dr. Paul Janssen Award winner, delivers his acceptance speech along with a discussion of the value of interesting vs. useful research in both academia and industry. “One of the reasons this award is meaningful and unique is because it recognizes the dual importance of academic research and pharmaceutical research, and the crucial and often quite difficult task of transforming interesting science into useful products for patients.” Watch the video above to hear more of Dr. Vogelstein’s insights."},{"id":32,"title":"Propelling Science, Transforming Oncology Research: Honoring Bert Vogelstein, M.D., Winner of the 2015 Dr. Paul Janssen Award for Biomedical Research","image":"/images/Bill-Hait-blogjpg.png","blogimageheight":700,"blogimagewidth":850,"blogimage":"/images/Bill-Hait-blogjpg_blog.png","date":"April 2016","videolink":"","videolink2":"","question":"","summary":"$14"},{"id":33,"title":"Why Prizes?","image":"/images/Award1.jpg","blogimageheight":700,"blogimagewidth":850,"blogimage":"/images/Award1_blog.jpg","date":"April 2016","videolink":"","videolink2":"","question":"","summary":"$15"},{"id":34,"title":"Celebrating the Dual Importance of Interesting and Useful Science Show More","image":"/images/2_Dual_Importance.jpg","blogimageheight":700,"blogimagewidth":850,"blogimage":"/images/2_Dual_Importance_blog.jpg","date":"April 2016","videolink":"","videolink2":"","question":"","summary":"$16"},{"id":35,"title":"Teaching Innovation","image":"/images/Rebecca-blog.png","blogimageheight":700,"blogimagewidth":850,"blogimage":"/images/Rebecca-blog_.png","date":"April 2016","videolink":"","videolink2":"","question":"","summary":"$17"},{"id":36,"title":"CRAIG C. MELLO, M.D.","image":"/images/Craig Mello - Portrait of Innovation.jpg","blogimageheight":700,"blogimagewidth":850,"blogimage":"/images/Craig Mello - Portrait of Innovation.jpg","date":"April 2016","videolink":"","videolink2":"","question":"","summary":"$18"},{"id":37,"title":"DAVID JULIUS, PH.D.","image":"/images/David-Julius-1301x603-no-logo.jpg","blogimageheight":700,"blogimagewidth":850,"blogimage":"/images/David-Julius-1301x603-no-logo.jpg","date":"April 2016","videolink":"","videolink2":"","question":"","summary":"$19"},{"id":38,"title":"BERT VOGELSTEIN, M.D.","image":"/images/BertVogelstein_HomepageImage_0.jpg","blogimageheight":700,"blogimagewidth":850,"blogimage":"/images/BertVogelstein_HomepageImage_0.jpg","date":"April 2016","videolink":"","videolink2":"","question":"","summary":"$1a"}]}]}]}]],null],null]},["$","$L1b",null,{"parallelRouterKey":"children","segmentPath":["children","newsroom","children"],"error":"$undefined","errorStyles":"$undefined","errorScripts":"$undefined","template":["$","$L1c",null,{}],"templateStyles":"$undefined","templateScripts":"$undefined","notFound":"$undefined","notFoundStyles":"$undefined","styles":null}],null]},[["$","html",null,{"lang":"en","children":["$","body",null,{"className":"__className_1585e8","children":[["$","$L1d",null,{}],["$","$L1b",null,{"parallelRouterKey":"children","segmentPath":["children"],"error":"$undefined","errorStyles":"$undefined","errorScripts":"$undefined","template":["$","$L1c",null,{}],"templateStyles":"$undefined","templateScripts":"$undefined","notFound":[["$","title",null,{"children":"404: This page could not be found."}],["$","div",null,{"style":{"fontFamily":"system-ui,\"Segoe UI\",Roboto,Helvetica,Arial,sans-serif,\"Apple Color Emoji\",\"Segoe UI Emoji\"","height":"100vh","textAlign":"center","display":"flex","flexDirection":"column","alignItems":"center","justifyContent":"center"},"children":["$","div",null,{"children":[["$","style",null,{"dangerouslySetInnerHTML":{"__html":"body{color:#000;background:#fff;margin:0}.next-error-h1{border-right:1px solid rgba(0,0,0,.3)}@media (prefers-color-scheme:dark){body{color:#fff;background:#000}.next-error-h1{border-right:1px solid rgba(255,255,255,.3)}}"}}],["$","h1",null,{"className":"next-error-h1","style":{"display":"inline-block","margin":"0 20px 0 0","padding":"0 23px 0 0","fontSize":24,"fontWeight":500,"verticalAlign":"top","lineHeight":"49px"},"children":"404"}],["$","div",null,{"style":{"display":"inline-block"},"children":["$","h2",null,{"style":{"fontSize":14,"fontWeight":400,"lineHeight":"49px","margin":0},"children":"This page could not be found."}]}]]}]}]],"notFoundStyles":[],"styles":null}],["$","main",null,{"className":"__className_1585e8","children":[["$","div",null,{"className":"text-center bg-gray-200 min-h-40 py-4 items-center","children":["$","h2",null,{"className":"__className_6a3843 text-3xl py-12","children":"Nominations are now closed"}]}],["$","div",null,{"className":"custom-footer min-h-40 bg-[#EB1700]","children":["$","div",null,{"className":"container mx-auto py-8 text-white text-sm","children":[["$","$L1e",null,{"src":"/images/JNJLogo_WhiteTransparent.png","width":240,"height":240,"unoptimized":true,"alt":"celebrate"}],["$","div",null,{"className":"flex flex-row pt-4 min-h-32","children":["$","div",null,{"className":"basis-1/2 ps-4","children":["$","p",null,{"children":["Dr. Paul Janssen Award Administration ",["$","br",null,{}],"Attn: Communications Department ",["$","br",null,{}],"920 Route 202 South ",["$","br",null,{}],"Raritan, NJ 08869, USA ",["$","br",null,{}],["$","a",null,{"className":"hover:underline decoration-2","target":"_blank","href":"mailto:RA-JJCUS-PJAward@corus.jnj.com","children":"RA-JJCUS-PJAward@corus.jnj.com"}]]}]}]}],["$","div",null,{"className":"min-h-6","children":["$","ul",null,{"className":"flex md:space-x-8 ps-4 pt-8","children":[["$","li",null,{"children":["$","$L1f",null,{"className":"hover:text-base","href":"/privacy","children":"Privacy Policy"}]}],["$","li",null,{"children":["$","$L1f",null,{"className":"hover:text-base","href":"/terms","children":"Legal Notice"}]}],["$","li",null,{"children":["$","$L1f",null,{"className":"hover:text-base","href":"/newsroom","children":"Newsroom"}]}],["$","li",null,{"children":["$","a",null,{"className":"hover:text-base","target":"_blank","href":"https://privacyportal.onetrust.com/webform/96f23ee1-34e3-41d6-8d5a-07f0d554152b/1c093578-7b73-4338-8629-baaf850965fc","title":"","children":"Do Not Sell or Share My Personal Information"}]}]]}]}]]}]}]]}]]}]}],null],null],[[["$","link","0",{"rel":"stylesheet","href":"/_next/static/css/81222508cbb8bc28.css","precedence":"next","crossOrigin":"$undefined"}],["$","link","1",{"rel":"stylesheet","href":"/_next/static/css/ee37ca26fb94f5d1.css","precedence":"next","crossOrigin":"$undefined"}]],"$L20"]]]]
20:[["$","meta","0",{"name":"viewport","content":"width=device-width, initial-scale=1"}],["$","meta","1",{"charSet":"utf-8"}],["$","title","2",{"children":"Dr. Paul Janssen Award"}],["$","meta","3",{"name":"description","content":"Generated by create next app"}],["$","link","4",{"rel":"icon","href":"/favicon.ico","type":"image/x-icon","sizes":"16x16"}],["$","meta","5",{"name":"next-size-adjust"}]]
1:null